Methods for Predicting Transonic Flutter Using Simple Data Models
aerospace
Methods for Predicting Transonic Flutter Using Simple Data Models (TOP2-313)
Resolving Pitching Airfoil Transonic Aerodynamics by Computational Fluid Dynamics (CFD) Data Modeling
Overview
Aircraft moving at transonic speeds (i.e., ~0.7 to 0.85 Mach - or near the speed of sound) experience transonic wing flutter. Engineers have traditionally relied on experimental or computational methods to understand wing flutter for the design process. Modeling wing flutter using the customary computational methods require tens of hours of simulations on a supercomputer that are costly to buy or rent. Having a method to model wing flutter aerodynamics without requiring supercomputer use would (a) increase the efficiency and decrease the cost of aircraft wing design and (b) enable real-time wing-flutter modeling to aid in-flight aircraft operation and control. Ames Research Center has developed a novel closed-form solution to model wing flutter aerodynamics for any aircraft wing (within a certain thickness regime and without camber). This closed-form solution can be readily extended to wing sections with camber. The closed-form solution matches, in near real-time, the complex supercomputer simulations at a fraction of cost and time necessary to perform transonic flutter modeling for an airfoil.
The Technology
Transonic flutter is a pacing item in transport aircraft design in that it is crucial to characterize this phenomenon for each aircraft to prevent catastrophic failure. Aerodynamic study of flows around airfoils is a canonical problem that entails both experimental and computational approaches. While the transonic flutter prediction can be more accurate with high-fidelity Computational Fluid Dynamics (CFD) methods than with unsteady potential flow methods, the computational cost is high. Therefore, computationally efficient methods for transonic flutter prediction continue to be of high interest to the aircraft design community. NASA Ames has developed a novel method that eliminates the need for expensive calculations of aerodynamics of wing flutter, which typically takes tens of hours on a supercomputer. Such calculations are now replaced by machine-learning-based closed form solutions that provide the solution almost instantaneously. The technology presents a new approach to predict the flow around pitching NACA00 series airfoils. NACA airfoils are generally symmetric, and thus they do not possess camber. However, the invention can readily extend to wings with camber. This novel data modeling approach is orders of magnitude faster than the traditional CFD approach of predicting aerodynamic effects of transonic pitching airfoils. The data model is based on a subset of unsteady CFD simulations that train the model. The trained model then resolves the pitching airfoil in time for any other set on the order of a second, as compared with a complete CFD simulation that typically takes 30 hours on a supercomputer. The data model is demonstrated in this invention for transonic flow corresponding to Mach number of 0.755 over pitching NACA00 series airfoils for a reduced frequency range typical of flutter, i.e., k lies in the range 0.02 - 0.25.
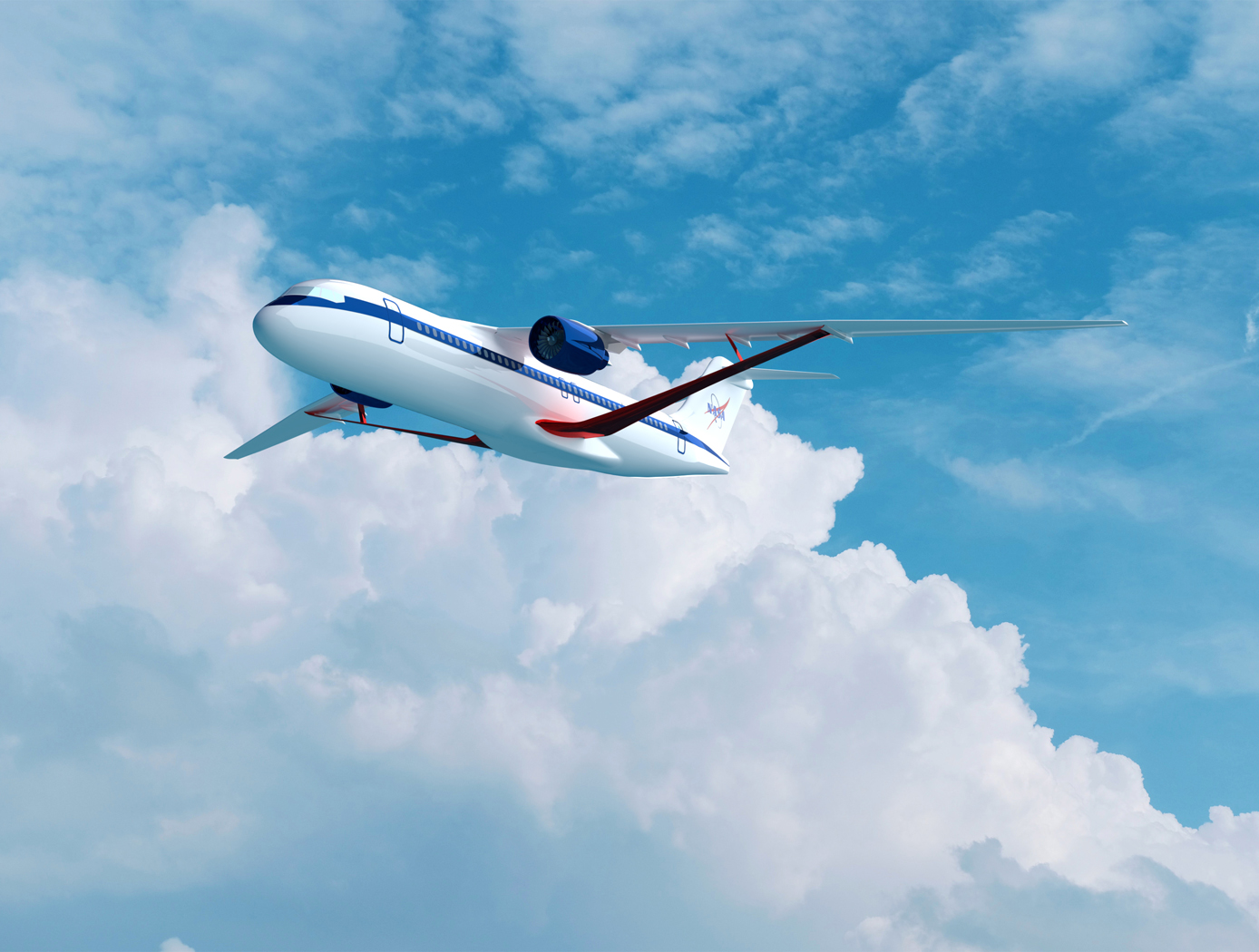
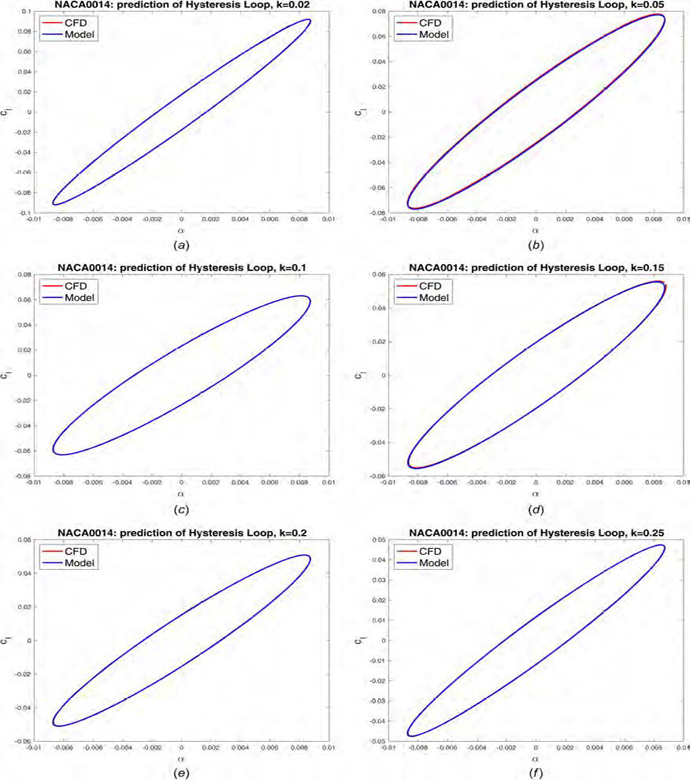
Benefits
- Decreases cost and complexity of wing design: eliminates the need for supercomputer modeling (which can cost tens of thousands of dollars per simulation)
- Enables real-time flight control: simplified, computationally efficient method of modeling wing flutter aerodynamics offers near real-time opportunities to let computations influence in-flight aircraft control
- Applies to existing and future aircraft: enables the implementation of active wing control systems to manage flutter on existing aircraft
- Applies to wing sections (airfoils) that are symmetric or cambered
- Less expensive and more efficient method to design an airfoil while taking flutter into account (method is based on a closed-form solution)
- Can be applied to aircraft in any Mach regime
Applications
- Aircraft wing design
- Active aircraft wing control
- Rotorcraft blades as they have similar characteristics to wings
- Can be applied directly to linear viscoelastic materials undergoing cyclic sinusoidal forces
Technology Details
aerospace
TOP2-313
ARC-18593-1
https://doi.org/10.1115/1.4050800