Search
Power Generation and Storage

Carbon Fiber Sleeve Tempers Battery Thermal Runaway
The CFRP sleeve was originally intended for crewed space flight lithium-ion 18650 battery packs rated over 80 Watt-hours (Wh), which are required to be passively propagation-resistant for increased safety. Previous battery designs have addressed SWR propagation by using aluminum or steel interstitial materials to prevent SWRs from directly impacting neighboring cells, but these materials were underperforming.
During testing of 18650 battery cells, it was discovered that cells over 2.6Ah in capacity can have an undesirable failure mode in which the cell wall will rupture or breach during a thermal runaway (TR) event sending heat and ejecta into an undesirable direction. TR is typically triggered when heat produced by the battery cell’s exothermic reaction leads to increased and escalating internal cell temperature, pressure, and boiling of the electrolytes. When internal cell pressure exceeds the cell’s safety relief mechanism, rupture or bursting can occur, initiating a cell-to-cell propagation that in turn results in a battery pack fire.
By adding a carbon fiber reinforced polymer (CFRP) sleeve to cylindrical battery cells, a sidewall rupture (SWR) can be prevented from occurring or propagating. In initial testing, there were no SWRs of a battery cell using a CFRP sleeve. This result is believed to be due in part to a unique characteristic of CFRP sleeves compared to other materials. Carbon fiber material has a negative coefficient of expansion and accordingly shrinks when heated, while steel and aluminum expand. The shrinking of the CFRP sleeve when heated compresses the cell located within it, significantly aiding in the prevention of SWR.
This technology can be implemented into other multi-physics battery safety models to guide the design of the next generation of battery cells and battery packs.
This thermal runaway propagation resistant technology has a technology readiness level (TRL) of 6 (System/sub-system model or prototype demonstration in an operational environment) and is now available for patent licensing. Please note that NASA does not manufacture products itself for commercial sale.
Power Generation and Storage
ThermoArc Facilitates Low-Cost Li-Ion Battery Testing
For years, NASA and the battery industry have been improving passive propagation resistant (PPR) Li-ion battery cell technology by enhancing their material and design choices. These efforts help ensure that a single cell’s TR event does not overheat adjacent cells or the entire battery pack ultimately causing fire or explosion. To improve cell integrity, single cells within battery packs are triggered into TR so that the battery pack can be analyzed for its TR resistance.
ThermoArc operates by initiating a plasma arc, capable of delivering thermal energy up to 100W, to a very small (1mm diameter) section of the cell. The extremely localized high heat flux rapidly degrades a small section of the internal cell separator, resulting in a short circuit that leads to TR. This technology comprises several components: a high-turn-ratio step-up transformer capable of producing a minimum of 1,000 V upon the secondary winding, an H-bridge electronic circuit to drive the transformer on the primary side, two tungsten electrodes to deliver the plasma arc, and a power supply unit.
ThermoArc applications may exist in any Li-ion battery cell/pack testing application where TR must be induced in an individual cell. Such applications could include testing of PPR battery packs to ensure single cell runaway does not cause catastrophic damage, more general battery destructive testing designed to better understand battery failure states, or other experimental testing. Companies interested in licensing this innovation may include those that manufacture internal short-circuit (ISC) cells or other devices used to induce TR at the individual cell level, battery testing firms, and Li-ion battery manufacturers with a focus on Li-ion battery packs for critical applications.
ThermoArc is at a technology readiness level (TRL) 5 (component and/or breadboard validation in laboratory environment) and is now available for patent licensing. Please note that NASA does not manufacture products itself for commercial sale.
Power Generation and Storage
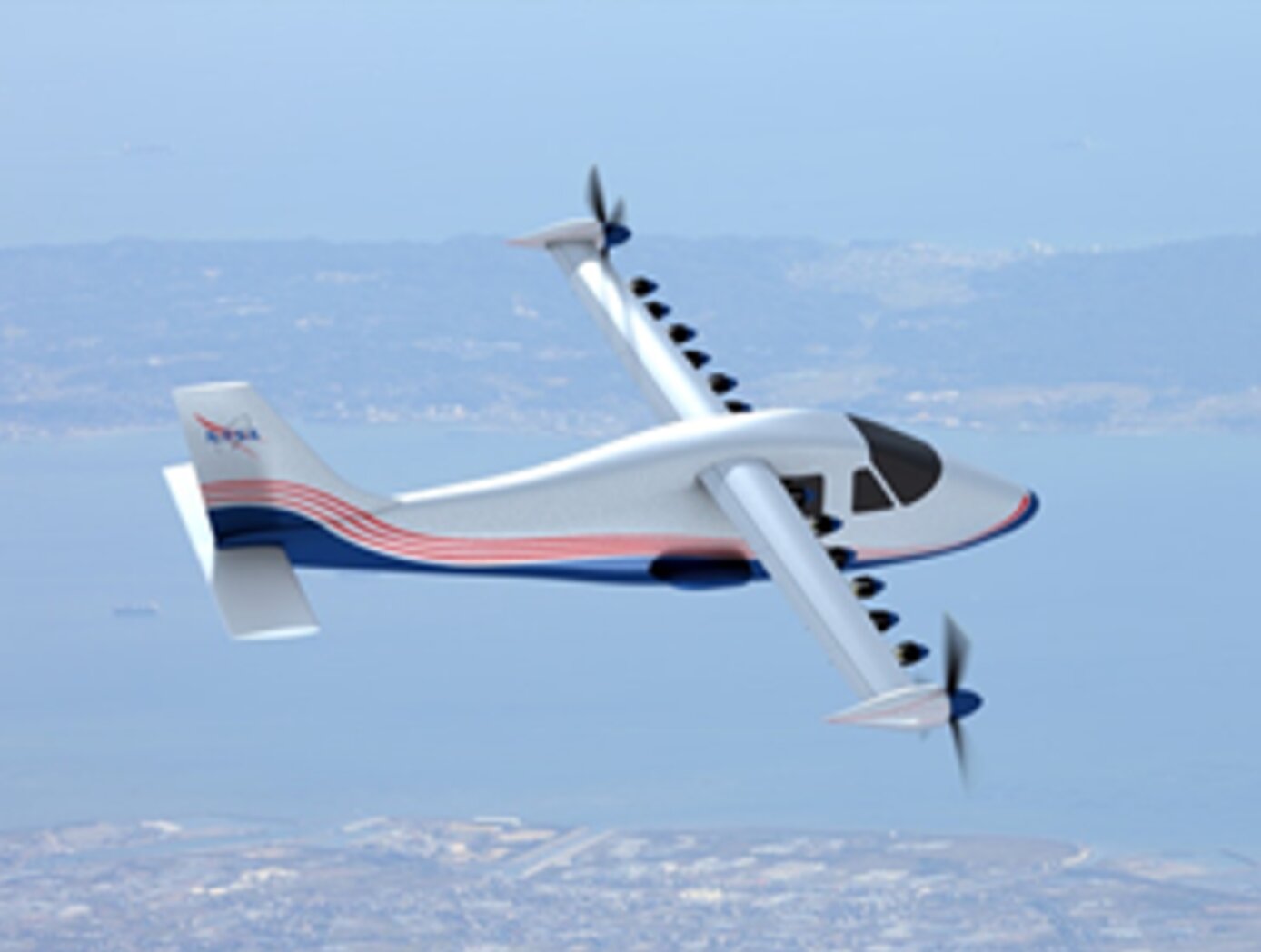
Solid-State Lithium-Sulfur Battery Tech Portfolio
The SABERS innovators developed novel lithium-sulfur designs, including sulfur-selenium on graphene cathodes, and lightweight bipolar plate stacking and packaging designs. SABERS is unique in several aspects: it deploys graphene-based manufacturing processes for the cathode and bipolar plates, and it uses a solid-state electrolyte in place of the liquid electrolyte found in other lithium-sulfur battery designs. The team has achieved energy densities over 500 W-hr/kg, and further improvements are expected. SABERS can meet the high-power requirements needed for aircraft take-off. SABERS is lightweight, safe, robust, and reliable. Furthermore, its manufacturing processes are scalable and environmentally friendly. Coin cell and pouch prototypes have been demonstrated to date. Development efforts continue and new portfolio innovations are expected. Major component technologies in SABERS include the following (as listed here and shown in the figure below). <ul>
<li>S/Se Cathode – Sulfur/Selenium on graphene scaffold
(LAR-19556-1, LEW-20228-1)
<li>Solid Electrolyte – Solid-state electrolyte composites
(LEW-20445-1)
<li>Bipolar Stack – Graphene plates
(LAR-20257-1)
<li>Li-Metal Anode
(Proprietary, under development)
<li>Packaging
(Proprietary, under development)</ul>
Robust computational models have been developed to support the battery materials design and are available to licensees to evaluate and optimize different materials combinations and performance targets.
Power Generation and Storage

Triggering Li-ion Battery Cells with Laser Radiation
This technology is based upon a 120-watt IR laser is coupled to a fiber optic cable that is routed from the output of the laser into a series of focusing optics which directs energy onto a battery cell mounted to a test stand. When activated, heat from the laser penetrates the metal housing, heating the internals of the cell. At a specific temperature, the separator in the first few layers of the cell melts allowing the anode and cathode to make contact and initiates an internal short circuit. The internal short circuit then propagates throughout the battery eventually causing thermal runaway. The lower the wavelength of the laser used to produce the thermal runaway, the more heat-energy will be absorbed into the cell producing a faster result. The fiber optic cable can be terminated into a series of optics to focus the laser at a specific target, or the fiber optic cable can be stripped bare and placed next to the target to heat an isolated location. This method can also be used on a wide variety of cells, including Li-ion pouch cells, Li-ion cylindrical cells and Li-ion Large format cells.
The innovation Triggering Li-ion Cells with Laser Radiation is at TRL 6 (which means a system/subsystem prototype has been demonstrated in a relevant environment) and the related patent application is now available to license and develop into a commercial product. Please note that NASA does not manufacture products itself for commercial sale.
Power Generation and Storage

Lunar Experiment Support System and Handling (LESSH) Battery Charger Module (BCM)
NASA’s LESSH BCM is a compact, high-performance, ruggedized system designed to support extended science operations in harsh lunar environments. With a mass of 9.4 kg and dimensions of 50 x 25 x 10 cm, the BCM is engineered for seamless integration with the interface bank on the HLS. A 1.5-meter flexible harness with an EVA-compatible connector and removable dust cover enables reliable operation in austere environments. Astronaut-operated controls, such as a guarded power switch and LED indicators, simplify usability and reduce the potential for errors during high-stakes lunar operations.
The BCM is optimized for safety and efficiency, incorporating state-of-the-art power and charging capabilities. It supports charging of 28V astronaut-rated batteries with a power output rated at 215W and integrates a battery pre-heater to maintain optimal performance in extreme temperatures. The BCM features a 4-hour charge time with software adjustability for charging parameters such as current, voltage, overvoltage, and undervoltage setpoints. Battery longevity is ensured through passive rebalancing of cell voltages and advanced safety features. Its 2-fault tolerant hardware and adjustable safety setpoints safeguard against potential hazards. Additionally, the BCM supports 1000BASE-T Ethernet pass-through for high-speed data transfer.
Originally designed to extend the length of lunar surface science experiments by enabling astronauts to recharge instrumentation, NASA’s LESSH BCM may be desired by companies seeking to operate sensors on the lunar or Martian lunar surface. The design may also be suitable for terrestrial applications involving harsh environments where interchangeable sealed sensors must operate on their own or with rovers, robotics, and drones. The BCM is at technology readiness level (TRL) 4 (component and/or breadboard validation in lab) and is available for patent licensing.
power generation and storage
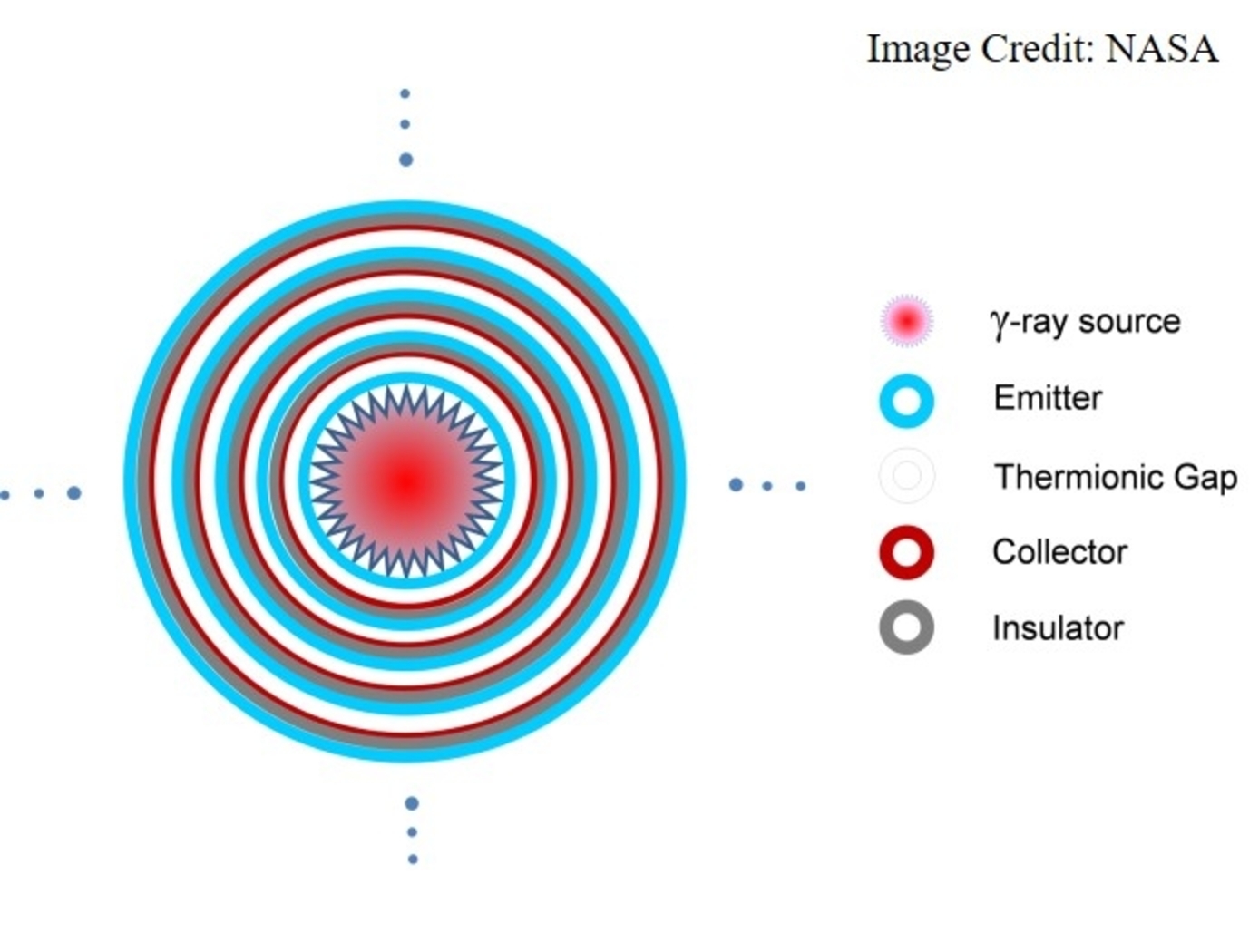
Multi-Layer Nuclear Thermionic Avalanche Cell
The Multi-Layer NTAC is comprised of a gamma-ray source and various layers of emitters, collectors, and insulators. Ideal emitter materials include elements with high atomic numbers, while ideal collector and insulator materials include elements with low atomic numbers. A high-energy gamma-ray (tens of keV to MeV) is used to liberate a large number of intra-band, inner-shell electrons from atoms within the emitter material for power generation through the primary interactions of photoelectric, Compton scattering, photonuclear, and electron/positron pair production processes. Secondary and tertiary electrons are liberated in the avalanche process as well. If a power conversion process effectively utilizes all liberated electrons in an avalanche mode through a power conversion circuit, the power output is drastically increased. Because power conversion is determined by the absorption rate of high energy photons, increasing power output requires either thicker collector material or a sufficient number of layer structures to capture the high energy photons, leaving no liberated electrons escaping (i.e., minimizing the leak of radioactive rays). The selection of materials, the thicknesses of the emitter, collector, and insulator, as well as the number of NTAC layers required are all determined by the energy of photon source. The thermal energy from radioactive decay can also be converted to electricity using a thermoelectric device to further increase power output. The Multi-Layer NTAC technology can be manufactured using existing semiconductor fabrication technology and can be tailored for small-to-large scale power needs, including kilowatt and megawatt applications.
Power Generation and Storage

Ram-Dent Thermal Runaway Triggering Device
The Ram-Dent Thermal Runaway Triggering Device is capable of trig-gering mild short circuits that are similar to internal defects, and more extreme short circuits that are comparable to those initiated by an implanted internal short circuit device – all without having to modify the battery cell in any way. The device imparts a high velocity, low mass blunt impact onto a small surface area of a Li-ion battery cell can wall allowing a small hemispherical dent to form. This impact deforms and tears the internal separator of the battery cell, causing a short circuit, and induces thermal runaway.
Several methods for initiating TR on demand currently exist, but they have characteristics and biases that make them more unfavorable for certain testing objectives. Heat-based insult in any form requires additional thermal energy to be applied to the battery cell, potentially distorting the signal-to-noise ratio in FTRC measurement, and increas-ing the severity of failure beyond what is intended. This methodology also requires time to heat the battery cell to critical temperature, and it potentially weakens the battery cell can wall causing abnormal kinetics and rupture. Mechanical insult methodologies, such as crush testing and nail penetration, have unique applications but rely on deformation of the battery cell itself which alters the flow characteristics of internal gases and the material integrity of the battery cell.
This technology aims to solve these limitations by implementing a cost-effective method to create a natural short circuit failure in Li-ion batteries that does not alter nominal vent paths and energy yields, potentially yielding the most unbiased calorimeter tallies yet.
The Ram-Dent Thermal Runaway Triggering Device is at a technology readiness level (TRL) 4 (component and/or breadboard validation in laboratory environment) and is now available for patent licensing. Please note that NASA does not manufacture products itself for commercial sale.
sensors
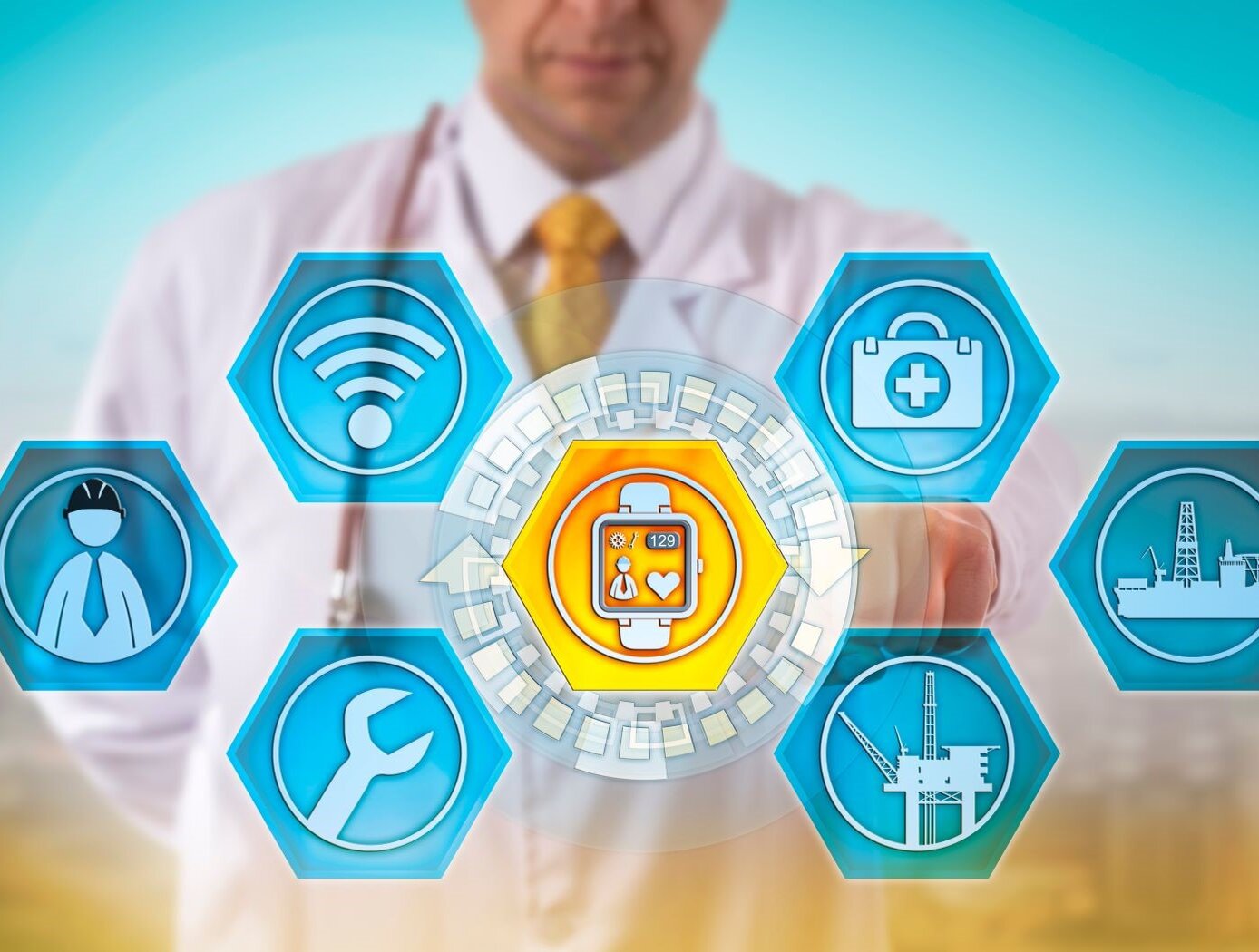
Wearable RFID Sensor Tags Yield Extended Operational Times
This technology exploits the inherently passive nature of RFID to approximate the services provided by traditional active Internet of Things (IOT) protocols like ZigBee and Bluetooth. A novel store-and-forward overlay on COTS RFID protocols allows an RFID active tags to transit through an ecosystem of RFID interrogators, exploiting contact opportunities as they arise and quietly transfers sensor readings at nearly no power cost to the RFID active tag. Specific intelligence built into both the interrogator and the tag leverages the RFID tag user memory (UM) as a stand-in IOT interface. The tag operates by sampling data into timestamped packets and loads them into tag memory. When an interrogator in the ecosystem realizes that a tag is in view and that there is unrecovered data on the tag, it takes custody of the sensor data packet and offloads the data into a database. A smart scheduler reads from the population of interrogators and schedules data transfers for specific tags when an interrogator can seed the custody transfer process for the data packets. NASA has produced working prototypes of wearables, worn by the crew aboard the International Space Station, that reports humidity, temperature and CO2 readings. In one estimate, the battery life is on pace to last an estimated nine years.
The Low-Power RFID to Collect and Store Data From Many Moving Wearable Sensors is a technology readiness level (TRL) 6 (system/subsystem prototype demonstration in a relevant environment). The innovation is now available for your company to license and develop into a commercial product. Please note that NASA does not manufacture products itself for commercial sale.
Optics
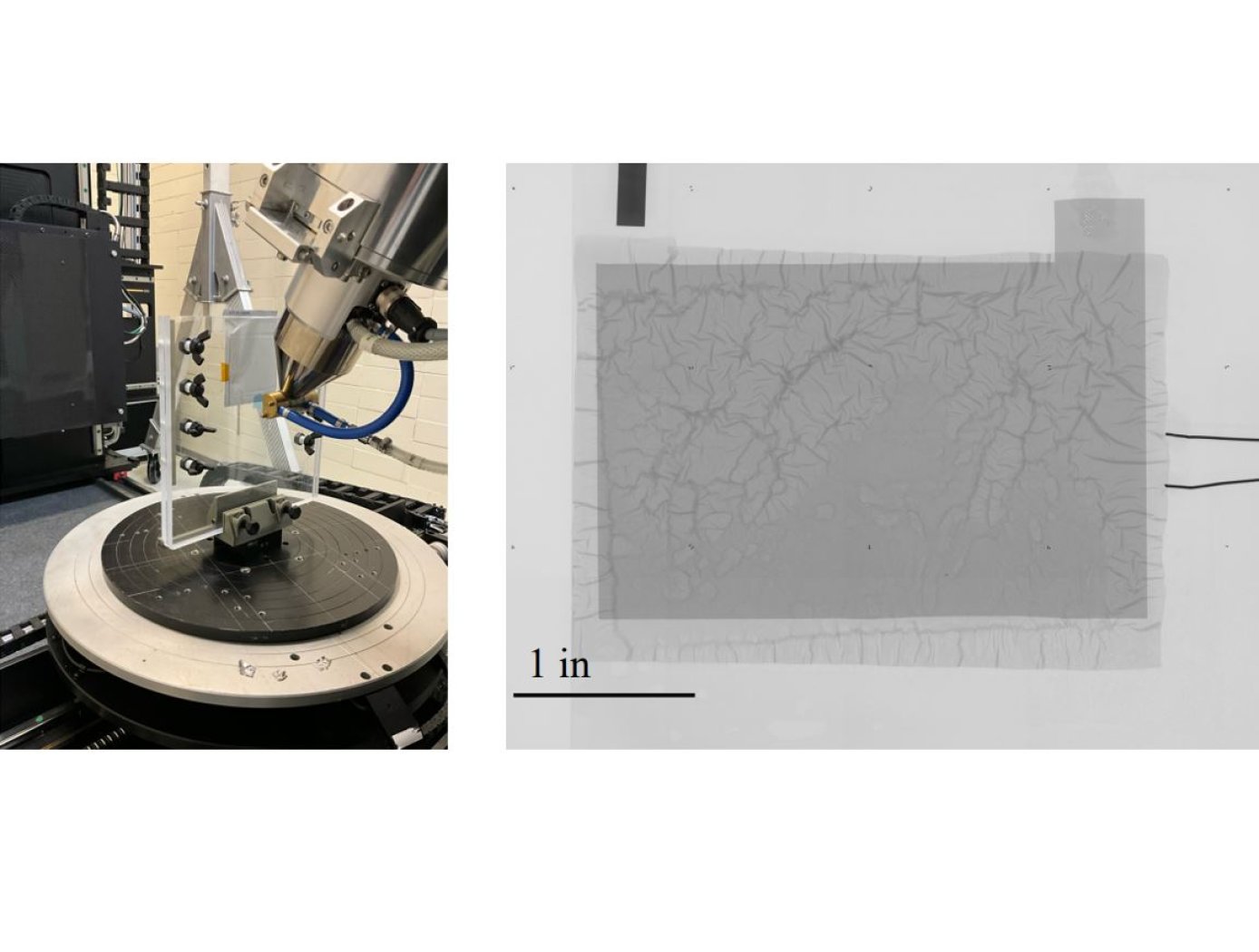
Fingerprinting for Rapid Battery Inspection
The technology utilizes photopolymer droplets (invisible to the digital radiograph) with embedded radiopaque fragments to create randomized fingerprints on battery samples. The droplets are deposited using a jig (see figure on right) that precisely positions samples. Then, at different points during battery R&D testing or use, digital radiography imaging with micron-level resolution can be performed.
The high-resolution imaging required to detect dendrite formation requires images to be collected in multiple “tiles” as shown below. The randomized fingerprints uniquely identify relative positioning of these tiles, allowing rapid assembly of composite high-resolution images from multiple tiles.
This same composite creation process can be used for images taken at a series of points in time during testing, and background subtraction can be applied to efficiently compare how the battery is changing over successive charge/discharge cycles to identify dendrite formation. This inspection technique is proven effective for thin-film pouch cell prototypes at NASA, and it works well at the lowest available x-ray energy level (limiting impact on the samples). The Fingerprinting for Rapid Battery Inspection technology is available for patent licensing.
Power Generation and Storage
Nail Penetration Adapter for
Li-Ion Battery Testing
NASA’s pneumatic nail penetration trigger system embodies a fast and consistent nail penetration and retraction tool that tests battery cells within various steel and mylar enclosures. It is operated remotely with electric power and shop air pressure inputs. The trigger system is controlled by a solenoid valve and drives a nail to a set distance at 100 m/s for a precise and repeatable penetration injury to a battery cell.
Contributing to the trigger system’s precision and repeatability is the nail penetration adapter that aligns the battery cell test enclosure with the trigger system and guides the nail by its internal barrel. The tip of the adapter threads into a variety of NASA battery cell test enclosures and provides an internal seating stop to ensure a proper targeting depth. On the actuator side of the adapter, two quick-release pins connect the adapter to the actuator mount which is bolted to the actuator subassembly. Removal of these pins readily allow for separation of the nail penetration adapter from the actuator mount and subassembly. During a TR event, the adapter is also designed to prevent flames, sparks, and ejecta from traveling into the actuator subassembly.
While the versatile nail penetration adapter was originally configured to interface with NASA’s Fractional Thermal Runaway Calorimeter (FTRC), a blast plate test platform (BPTP), or cell enclosure for passive propagation resistant (PPR) methods, the adapter could be modified for commercial use with other battery testing systems. Companies interested in this technology may include those seeking to improve the safety of Li-ion battery cells and packs along with vertically integrated companies performing in-house TR tests during development of electrified systems like electric vehicles (EVs), electric vertical takeoff and landing (eVTOLs) vehicles, and electronic spacecraft components.