Search
Instrumentation
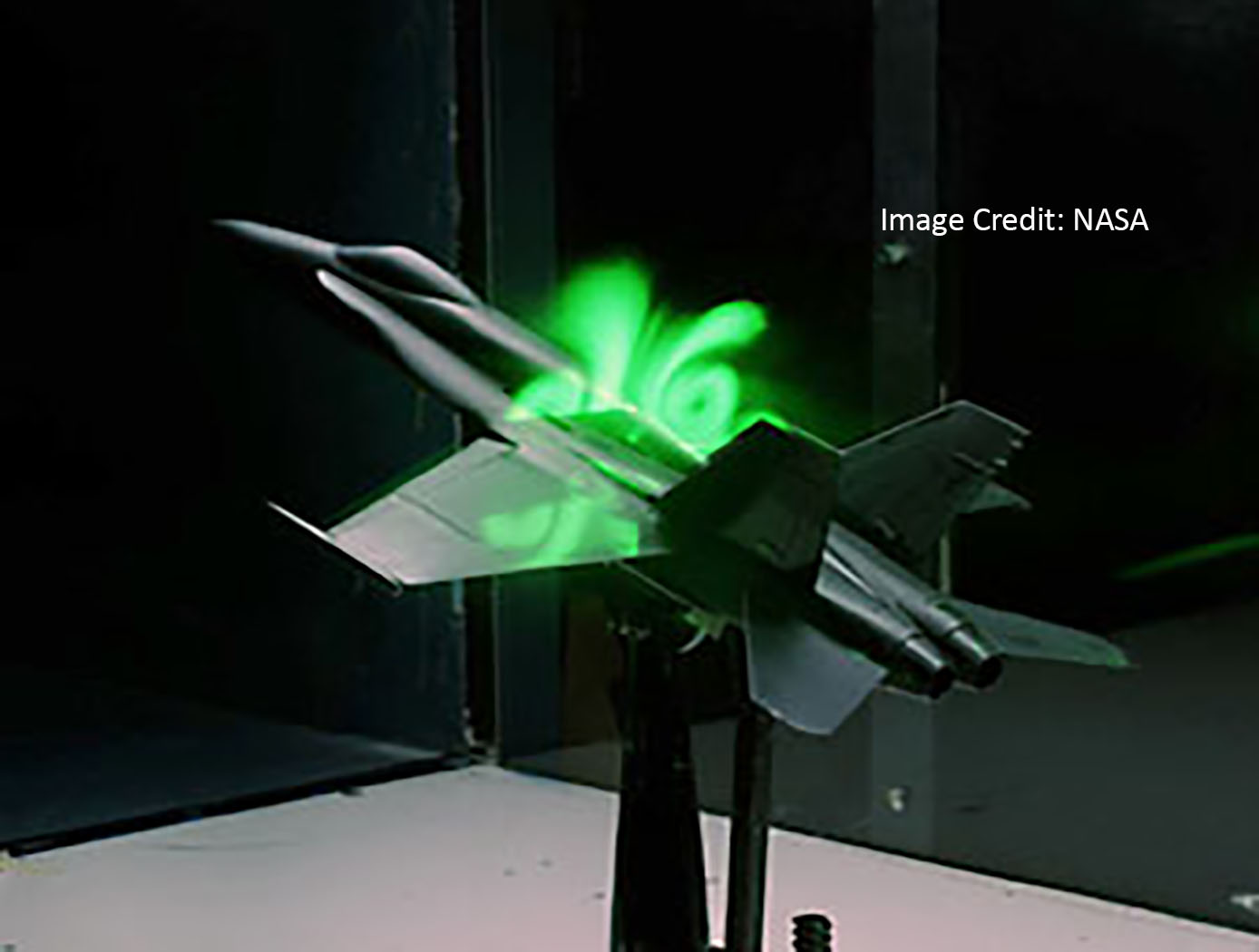
Generation of Polystyrene Latex Spheres with Incorporated Fluorescent Dyes
Although polystyrene microspheres are often the seed material of choice for subsonic airflow studies. These seed materials, however, do not provide any benefit for near wall measurements compared to other state-of-the-art seed materials. Consequently, in this innovation NASA scientists have developed this method of generating dye-doped polystyrene microspheres using novel synthetic approaches.
The novel features of this invention are the utility of specific chemical functionalities, monomeric species, environmental additives (buffers), and polyelectrolytes to promote incorporation of dye molecules into developing polystyrene microspheres while enabling control of the spectral properties of the dye relative to pH dependence. These particles will have great utility for wind tunnel measurements near the wall where the state-of-the-art seed materials are not able to collect data. Additionally, the incorporation of these dyes will offer other avenues of data collection including temperature and pressure of the airflows and wind tunnel regions. Likewise, the ability to selectively filter the data collected from these dye-doped polystyrene microspheres can have further applications including the direct visualization of 2 or more fluid flows mixing, among other applications.
instrumentation
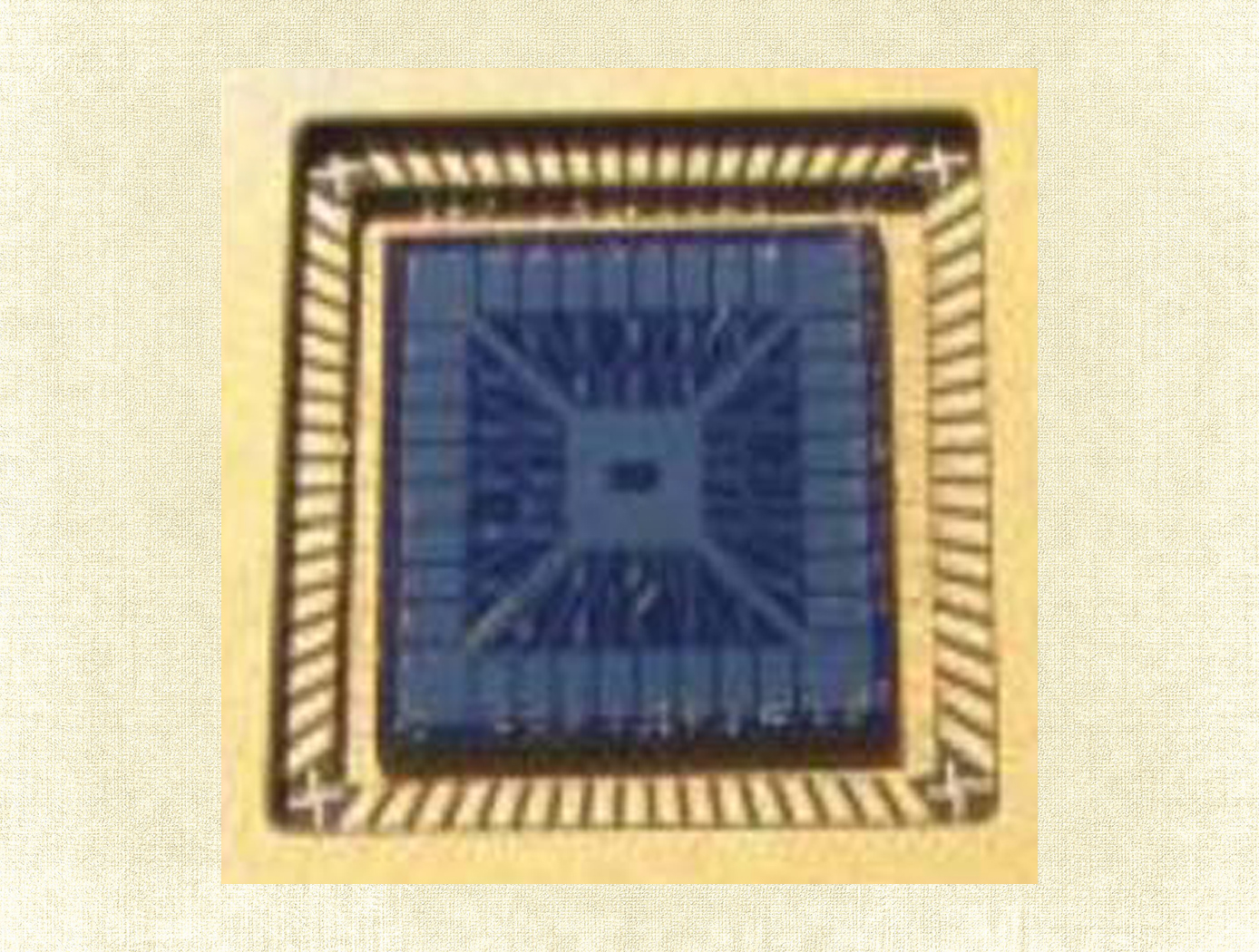
Portable Medical Diagnosis Instrument
The technology utilizes four cutting-edge sensor technologies to enable minimally- or non-invasive analysis of various biological samples, including saliva, breath, and blood. The combination of technologies and sample pathways have unique advantages that collectively provides a powerful analytical capability. The four key technology components include the following: (1) the carbon nanotube (CNT) array designed for the detection of volatile molecules in exhaled breath; (2) a breath condenser surface to isolate nonvolatile breath compounds in exhaled breath; (3) the miniaturized differential mobility spectrometer (DMS) -like device for the detection of volatile and non-volatile molecules in condensed breath and saliva; and (4) the miniaturized circular disk (CD)-based centrifugal microfluidics device that can detect analytes in any liquid sample as well as perform blood cell counts. As an integrated system, the device has two ports for sample entry a mouthpiece for sampling of breath and a port for CD insertion. The breath analysis pathway consists of a CNT array followed by a condenser surface separating liquid and gas phase breath. The exhaled breath condensate is then analyzed via a DMS-like device and the separated gas breath can be analyzed by both CNT sensor array again and by DMS detectors.
Health Medicine and Biotechnology
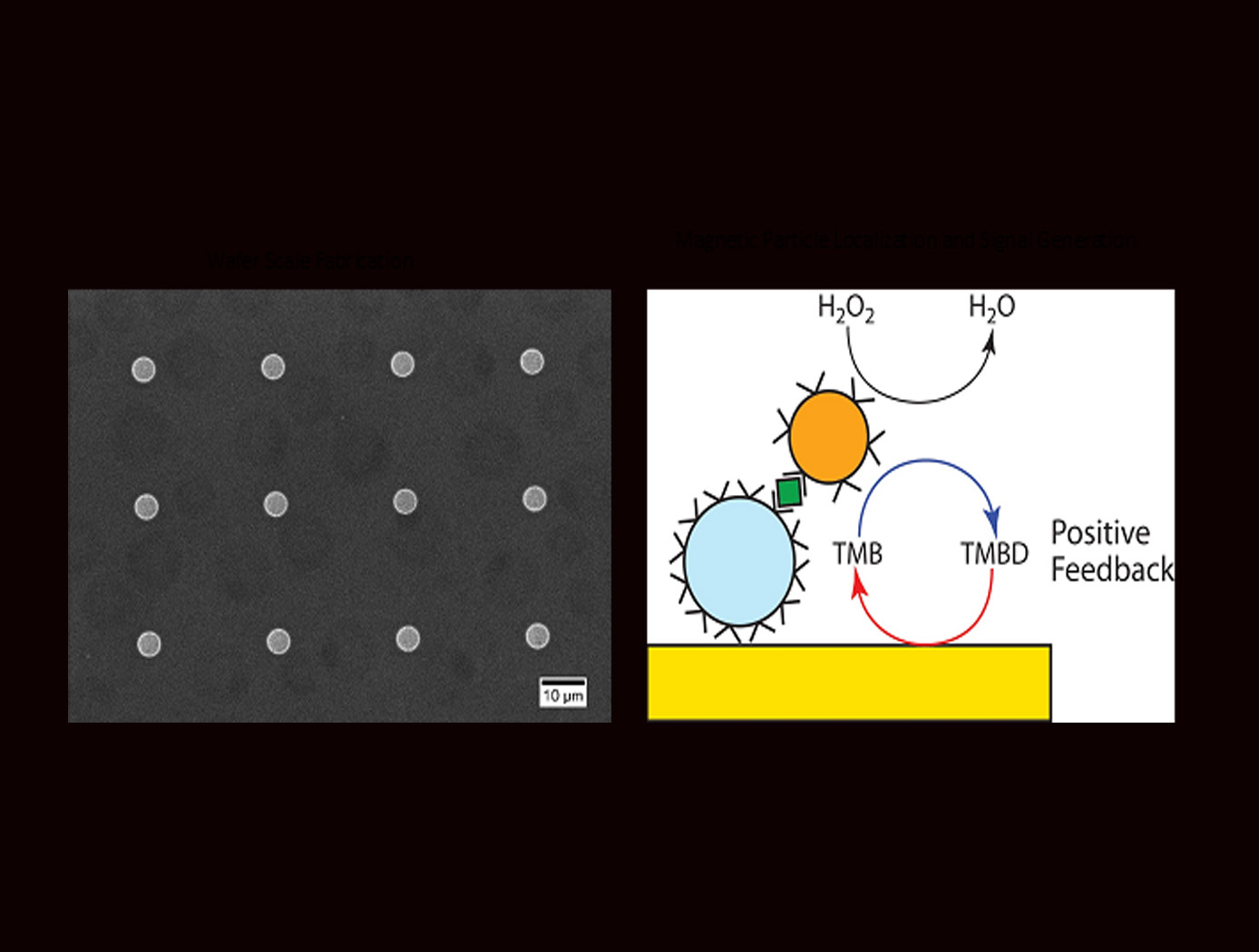
Electrochemical Sensors Based on Enzyme-Linked Immunosorbent Assay
NASA’s electrochemical Enzyme-Linked Immunosorbent Assay (ELISA) microelectrode array biosensor advantageously incorporates a microbead detection construct, coupled with a magnetic immobilization construct, which substantially increases the signal sensitivity of a sensor. The magnetic immobilization construct draws the microbead detection construct to an electrode detection surface, enhancing signal sensitivity. By concentrating the signaling molecules close to the electrode detection surface, electrochemical redox cycling is achieved by reducing the distance between the two, allowing for regeneration of reporter molecules.
Whereas a traditional ELISA testing exhibits five to ten signaling molecules per probe molecule binding event, the present electrochemical ELISA-based biosensor testing exhibits up to 4,857 signaling molecules per probe molecule binding event. The model bead construct exhibits a more than 6.75-fold in increased measured signal, and more than 35.7-fold improvement in signal sensitivity. When compared to traditional optical ELISA, the present invention improves the limit of detection by up to a factor of 60.5.
NASA’s electromagnetic ELISA-based biosensor can be used for the detection of SARS-CoV-2 virus to enhance Covid-19 testing during the early phases of infection. The technology may also be modified to detect other biomarkers.
propulsion

HYPERFIRE
In order to maintain the low cost, simplicity, and quick turnaround of cold-flow testing while improving accuracy, NASA evaluated unconventional gases for use as simulants. During such evaluations, NASA discovered that by adjusting stagnation temperature, the isentropic exponent of ethane can be tuned to approximate those of common rocket propellants (e.g., hydrogen, hypergols, alcohols, and hydrocarbons). Furthermore, due to ethanes high auto-ignition temperature and resistance to condensation, tuned ethane enables testing of expansion ratios much larger than conventional inert-gas testing.
To leverage this discovery, NASA developed a hardware-based system to treat ethane and obtain nozzle chamber conditions that match the appropriate aerodynamics for a specific test. The system, named HYPERFIRE, works in the following manner. Liquid ethane is transferred to a piston-style run tank, where it is pressurized. Then, the ethane is run through two insulated pebble beds where it is heated, vaporized, and stabilized. Finally, the treated ethane is transferred from the second pebble bed to a small thrust takeout structure, and through the test article. Control of valves and regulators is managed by an onboard computer, accessed via a LabVIEW™ interface. The system is mounted on a hurricane-resistant steel frame to enable transportation via forklift.
Heated ethane reproduces the aerodynamics of combustion products at low temperatures relative to alternative testing methods. Thus, test articles can be manufactured using low-cost, low temperature rated, transparent materials (e.g., acrylic). In addition to reducing testing cost, this grants optical access to internal flowfields, enabling advanced diagnostic techniques (e.g., Schlieren imaging, particle image velocimetry) not possible with hot-fire testing and less meaningful with conventional cold-flow testing.
Aerospace
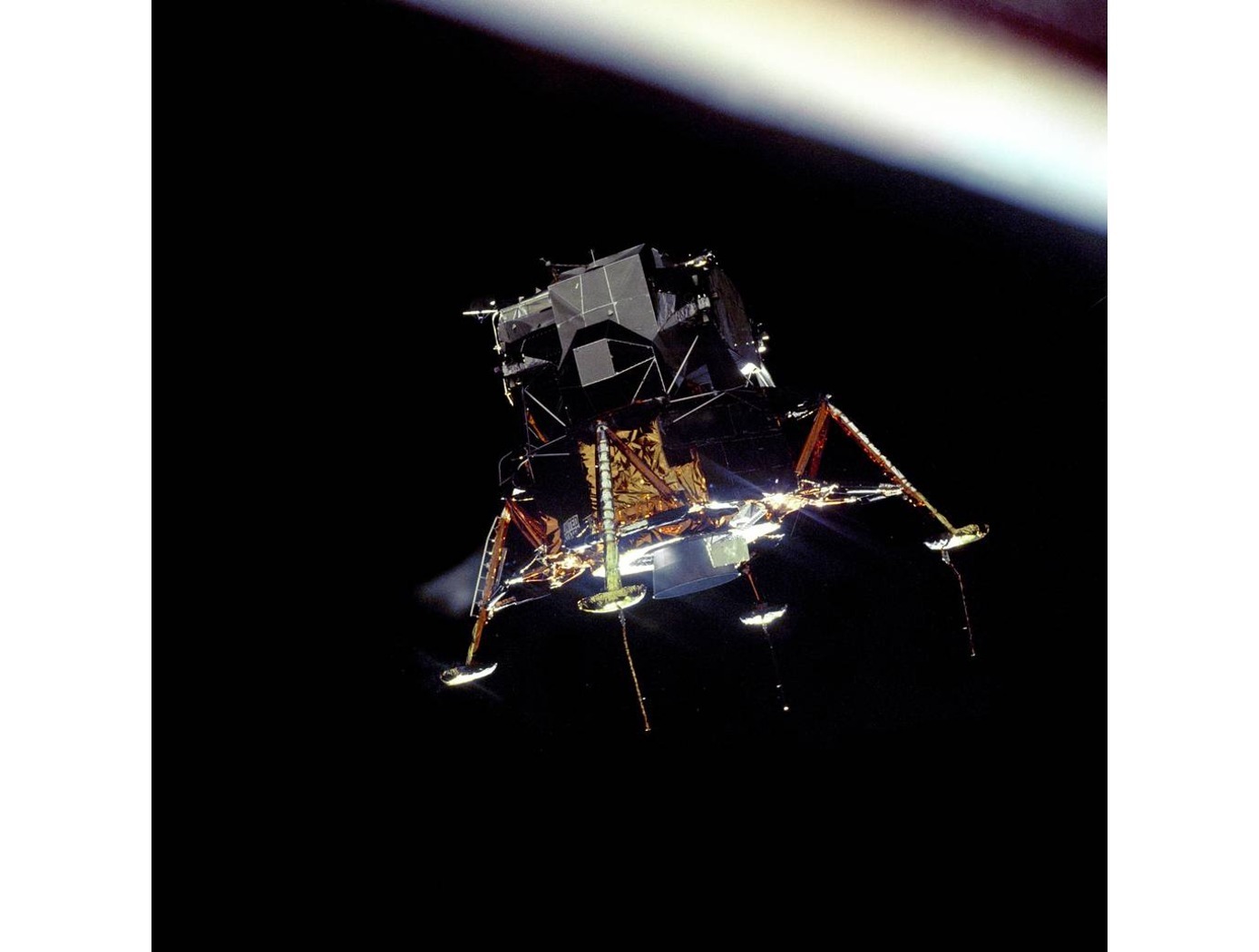
eVTOL UAS with Lunar Lander Trajectory
This NASA-developed eVTOL UAS is a purpose-built, electric, reusable aircraft with rotor/propeller thrust only, designed to fly trajectories with high similarity to those flown by lunar landers. The vehicle has the unique capability to transition into wing borne flight to simulate the cross-range, horizontal approaches of lunar landers. During transition to wing borne flight, the initial transition favors a traditional airplane configuration with the propellers in the front and smaller surfaces in the rear, allowing the vehicle to reach high speeds. However, after achieving wing borne flight, the vehicle can transition to wing borne flight in the opposite (canard) direction. During this mode of operation, the vehicle is controllable, and the propellers can be powered or unpowered.
This NASA invention also has the capability to decelerate rapidly during the descent phase (also to simulate lunar lander trajectories). Such rapid deceleration will be required to reduce vehicle velocity in order to turn propellers back on without stalling the blades or catching the propeller vortex. The UAS also has the option of using variable pitch blades which can contribute to the overall controllability of the aircraft and reduce the likelihood of stalling the blades during the deceleration phase.
In addition to testing EDL sensors and precision landing payloads, NASA’s innovative eVTOL UAS could be used in applications where fast, precise, and stealthy delivery of payloads to specific ground locations is required, including military applications. This concept of operations could entail deploying the UAS from a larger aircraft.
health medicine and biotechnology
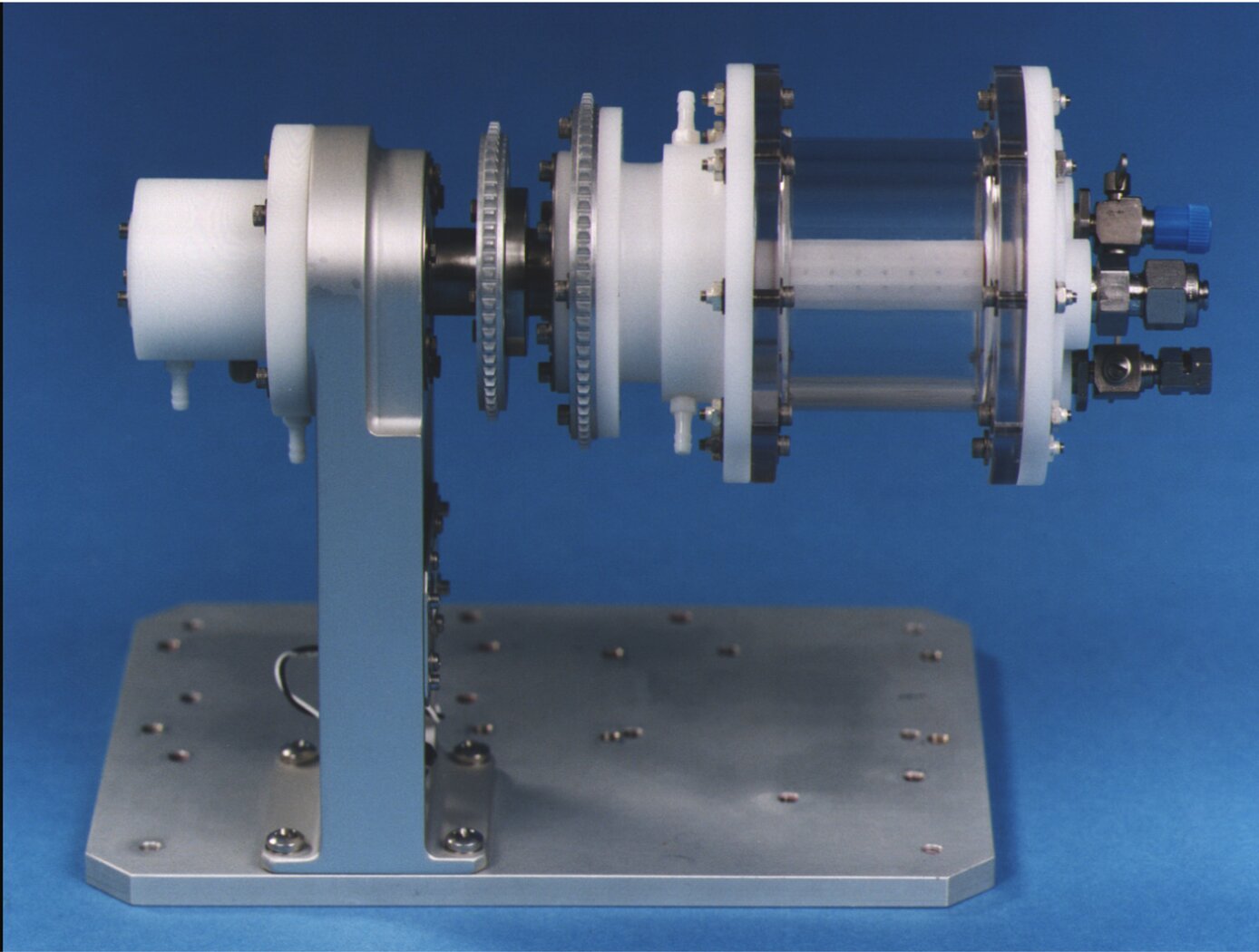
Human Tissue-Like Cellular Assemblies Grown for Respiratory Studies
In vitro three-dimensional (3D) human broncho-epithelial (HBE) tissue-like assemblies (3D HBE TLAs or TLAs) were engineered in modeled microgravity using rotating wall vessel technology (pictured above) to mimic the characteristics of in vivo tissue. The TLAs were bioengineered onto collagen-coated cyclodextran beads using primary human mesenchymal bronchial-tracheal cells (HBTC) as the foundation matrix and an adult human broncho-epithelial immortalized cell line (BEAS-2B) as the overlying component. The resulting TLAs share significant characteristics with in vivo human respiratory epithelium including polarization, tight junctions, desmosomes, and microvilli. The presence of tissue-like differentiation markers including villi, keratins, and specific lung epithelium markers, as well as the production of tissue mucin, further confirm these TLAs have differentiated into tissues functionally like in vivo tissues. TLAs mimic aspects of the human respiratory epithelium and provide a unique capability to study the interactions of respiratory viruses and their primary target tissue independent of the host's immune system.
The innovation "Methods For Growing Tissue-Like 3D Assemblies Of Human Broncho-Epithelial Cells" is at Technology Readiness Level (TRL) 6 (which means system/subsystem prototype demonstration in a relevant environment) and the related patent is now available to license for development into a commercial product. Please note that NASA does not manufacture products itself for commercial sale.
Electrical and Electronics
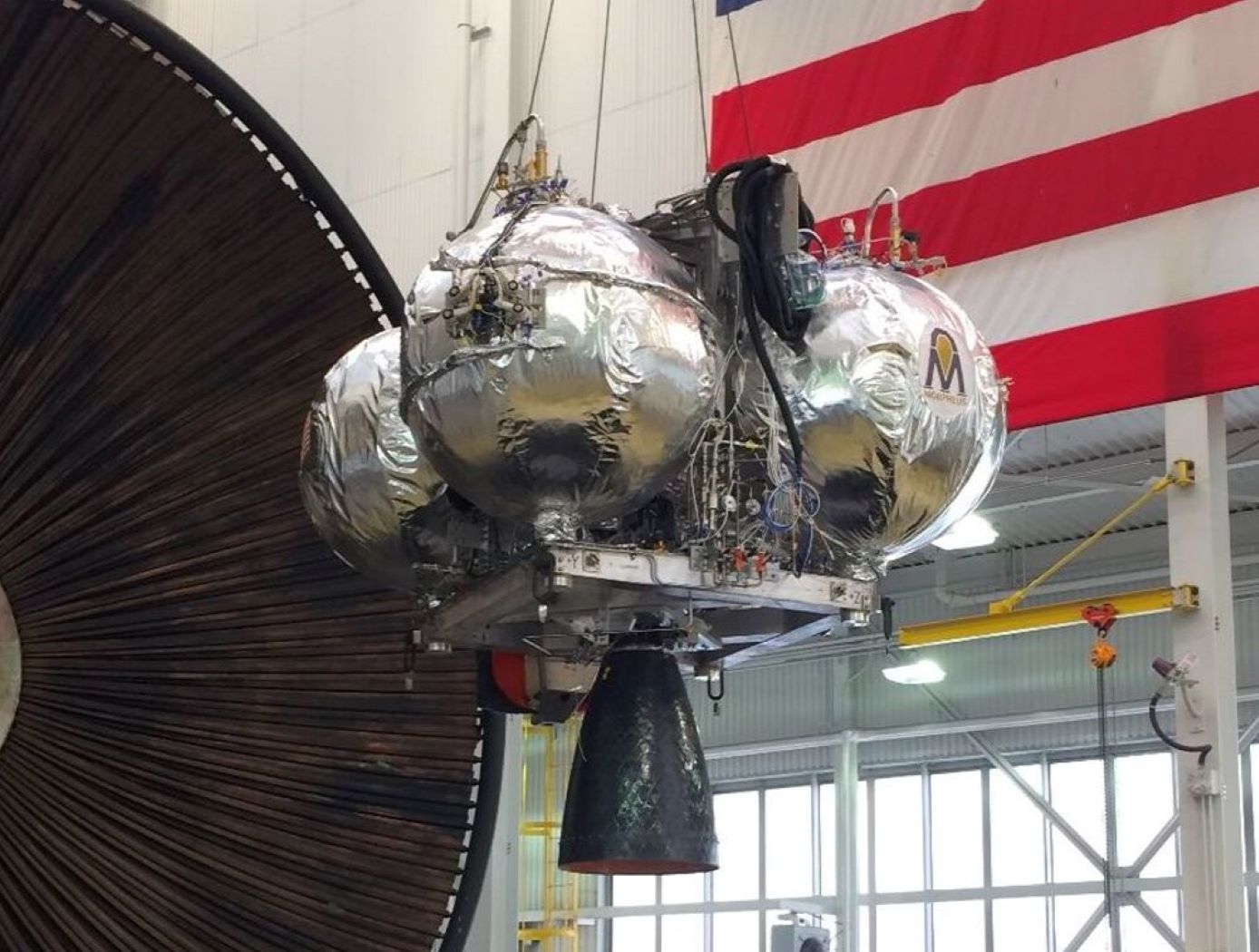
Coil-On-Plug Igniter for Reliable Engine Starts
Spark-ignition devices have proven to be a high-reliability option for LOX/LCH4 ignition during development of the Integrated Cryogenic Propulsion Test Article (ICPTA) main and reaction control engines (RCEs); however, issues including spark plug durability (ceramic cracking) and corona discharge during simulated altitude testing have been observed, contributing to degraded spark output and no-light engine-start conditions. Innovators discovered that ignition system reliability could be improved and weight reduced by eliminating the traditional coil and spark plug wire. To achieve this result, engineers made the innovation by modifying an automotive coil-on-plug igniter to provide new high sparking energies at the point of combustion using low supply voltages. The coil was modified by vacuum-potting it into a threaded interface that mounts into existing spark plug ports on the ICPTA main engine and the RCEs. Engineers fabricated custom electrode tips that were thread-mounted into the potted coil body. Epoxy insulation was chosen with high dielectric strength to maintain insulation between the electrode and threaded adapter. Vacuum potting successfully prevented pressure or vacuum leakage into the coil body and maintained spark energy and location at the electrode tip. Successful hot-fire ignition was observed at sea-level, altitude, and thermal-vacuum for both ICPTA RCE and main engine igniters down to 10^-3 torr, which approaches the vacuum of cislunar space.
This technology is at technology readiness level (TRL) 7 (system prototype demonstration in an operational environment), and the related patent is now available to license. Please note that NASA does not manufacture products itself for commercial sale.
manufacturing
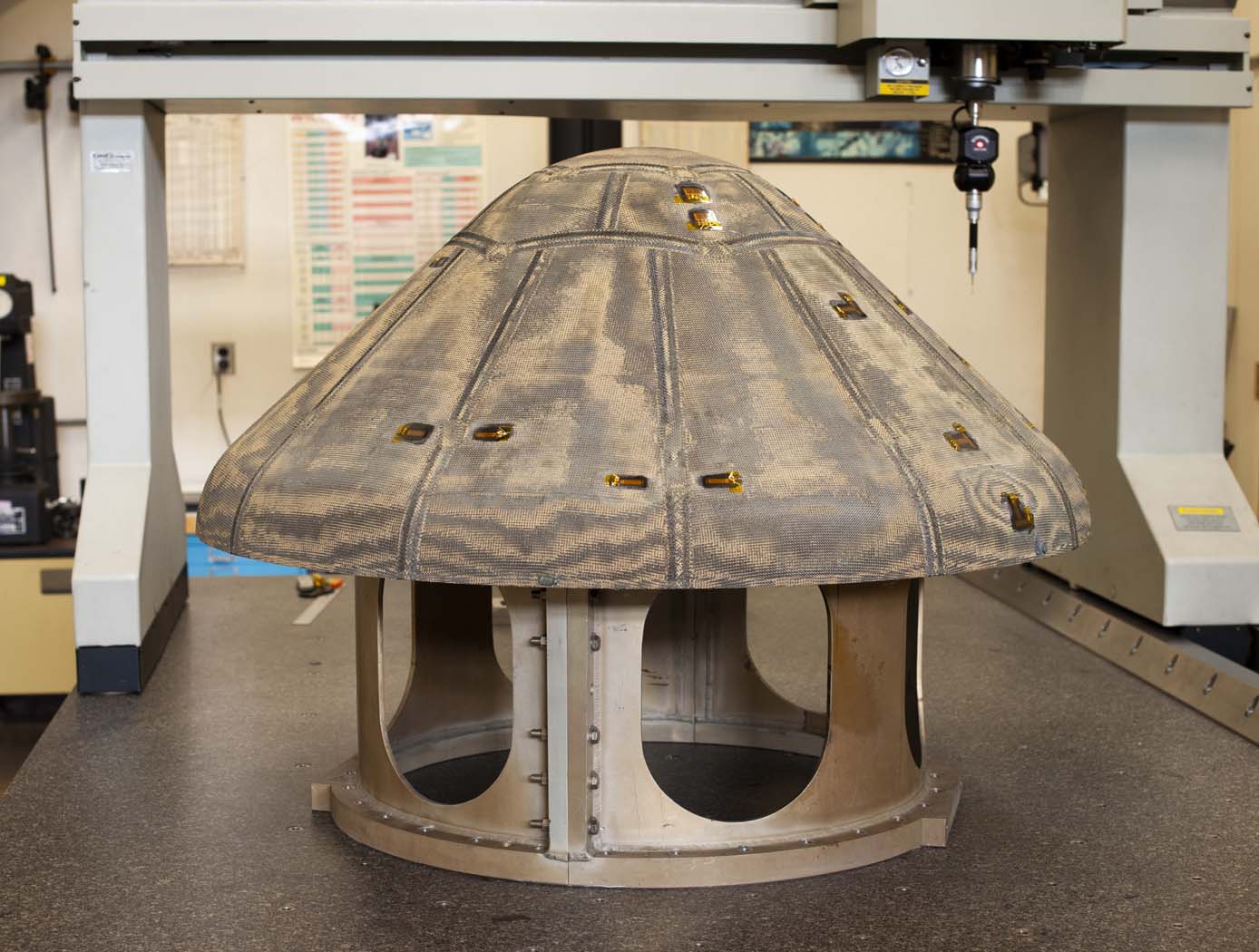
Woven Thermal Protection System
Going farther, faster and hotter in space means innovating how NASA constructs the materials used for heat shields. For HEEET, this results in the use of dual-layer, three-dimensional, woven materials capable of reducing entry loads and lowering the mass of heat shields by up to 40%. The outer layer, exposed to a harsh environment during atmospheric entry, consists of a fine, dense weave using carbon yarns. The inner layer is a low-density, thermally insulating weave consisting of a special yarn that blends together carbon and flame-resistant phenolic materials. Heat shield designers can adjust the thickness of the inner layer to keep temperatures low enough to protect against the extreme heat of entering an atmosphere, allowing the heat shield to be bonded onto the structure of the spacecraft itself. The outer and inner layers are woven together in three dimensions, mechanically interlocking them so they cannot come apart. To create this material, manufacturers employ a 3-D weaving process that is similar to that used to weave a 2-D cloth or a rug. For HEEET, computer-controlled looms precisely place the yarns to make this kind of complex three-dimensional weave possible. The materials are woven into flat panels that are formed to fit the shape of the capsule forebody. Then the panels are infused with a low-density version of phenolic material that holds the yarns together and fills the space between them in the weave, resulting in a sturdy final structure. As the size of each finished piece of HEEET material is limited by the size of the loom used to weave the material, the HEEET heat shield is made out of a series of tiles. At the points where each tile connects, the gaps are filled through inventive designs to bond the tiles together.
Manufacturing
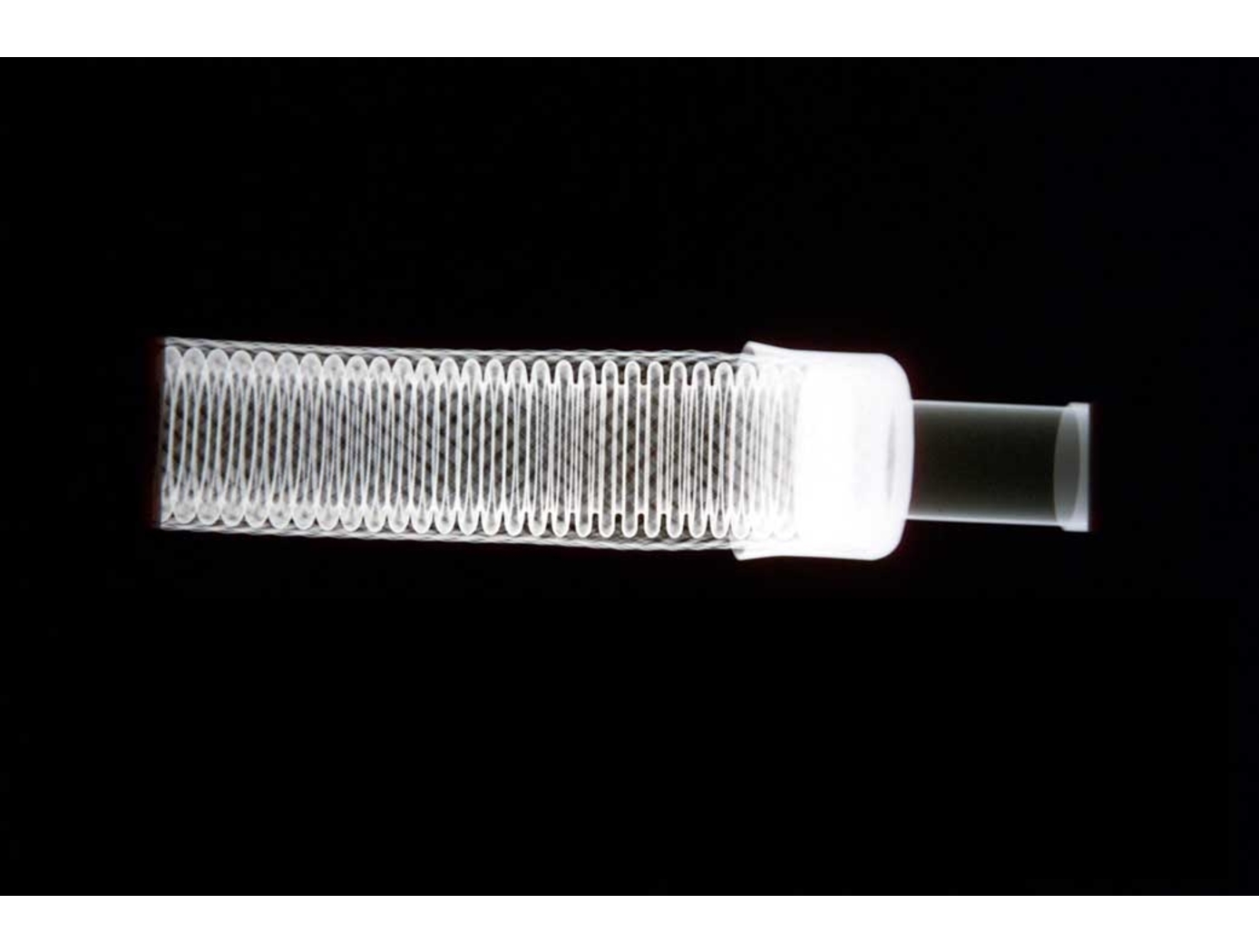
X-Ray Crack Detectability
NASAs software technology uses an Image Quality Indicator (IQI)-based model that can predict whether cracks of a certain size can be detected, as well as a model that can provide appropriate conditions to optimize x-ray crack detection setup. Because this modeling software can predict minimum crack sizes that can be detected by a particular X-ray radiography testing setup, users can test various setups until the desired crack detection capabilities are achieved (predicted) by the modeling system.
These flaw size parameter models use a set of measured inputs, including thickness sensitivity, detector modulation transfer function, detector signal response function, and other setup geometry parameters, to predict the minimum crack sizes detectable by the testing setup and X-ray angle limits for detecting such flaws.
Current X-ray methods provide adequate control for detection of volumetric flaws but do not provide a high probability of detection (POD), and crack detection sensitivity cannot be verified for reliable detection. This results in reduced confidence in terms of crack detection. Given that these cracks, if undetected, can cause catastrophic failure in various systems (e.g., pressure vessels, etc.), verifying that X-ray radiography systems used for NDE can detect such cracks is of the utmost importance in many applications.
Mechanical and Fluid Systems
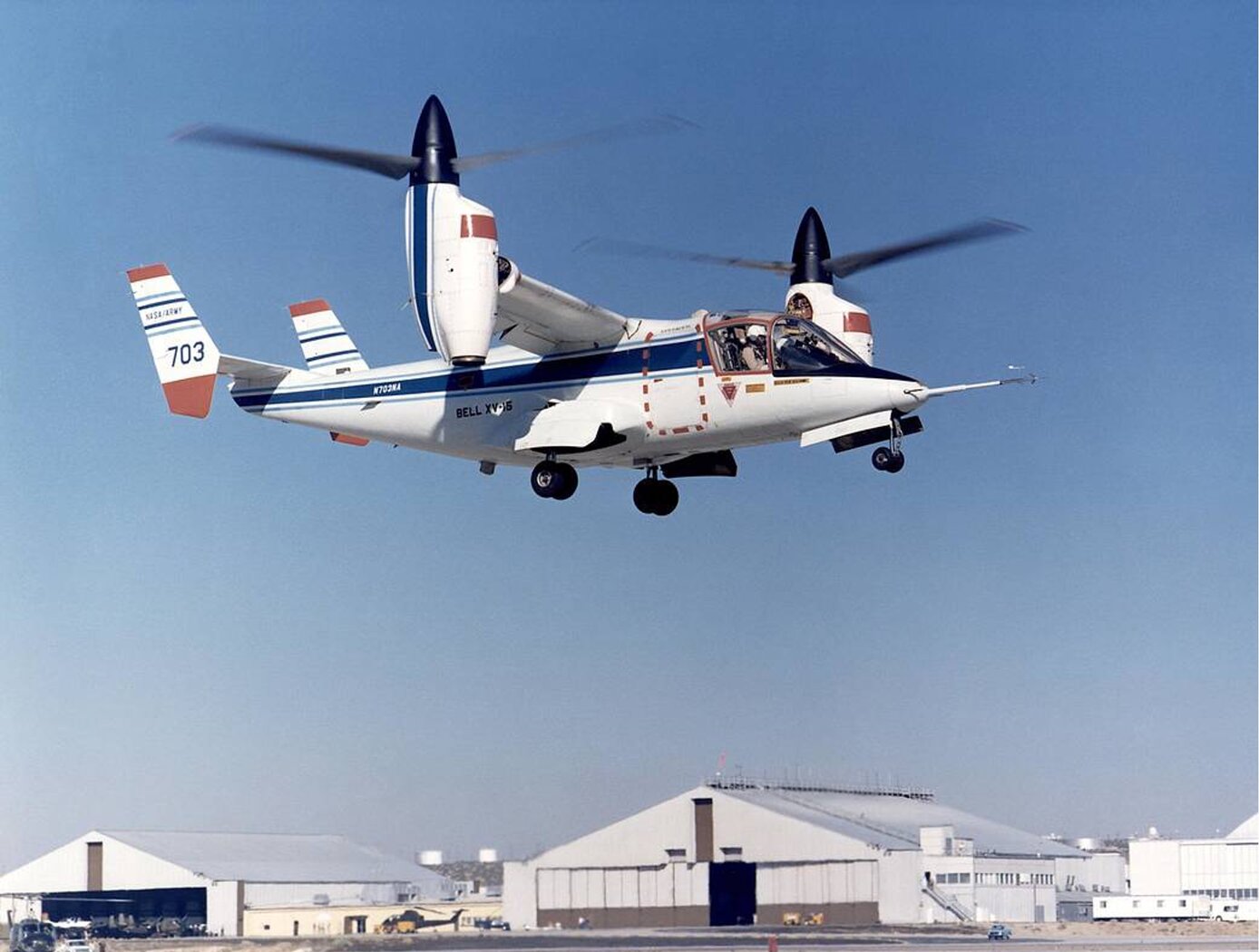
Improving VTOL Proprotor Stability
Proprotors on tiltrotor aircraft have complex aeroelastic properties, experiencing torsion, bending, and chord movement vibrational modes, in addition to whirl flutter dynamic instabilities. These dynamics can be stabilized by high-frequency swashplate adjustments to alter the incidence angle between the swashplate and the rotor shaft (cyclic control) and blade pitch (collective control). To make these high-speed adjustments while minimizing control inputs, generalized predictive control (GPC) algorithms predict future outputs based on previous system behavior. However, these algorithms are limited by the fact that tiltrotor systems can substantially change in orientation and airspeed during a normal flight regime, breaking system continuity for predictive modeling.
NASA’s Advanced GPC (AGPC) is a self-adaptive algorithm that overcomes these limitations by identifying system changes and adapting its predictive behavior as flight conditions change. If system vibration conditions deteriorate below a set threshold for a set time interval, the AGPC will incrementally update its model parameters to improve damping response. AGPC has shown significant performance enhancements over conventional GPC algorithms in comparative simulations based on an analytical model of NASA’s TiltRotor Aeroelastic Stability Testbed (TRAST). Research for Hardware-In-the-Loop testing and flight vehicle deployment is ongoing, and hover data show improved vibration reduction and stability performance using AGPC over other methods.
The example presented here is an application to tiltrotor aircraft for envelope expansion and vibration reduction. However, AGPC can be employed on many dynamic systems.