Double Sided Si(Ge)/Sapphire/III-Nitride Hybrid Structures
electrical and electronics
Double Sided Si(Ge)/Sapphire/III-Nitride Hybrid Structures (LAR-TOPS-161)
Method to produce combination of devices on opposite sides of a sapphire substrate
Overview
NASA Langley Research Center has developed a double sided Si(Ge)/Sapphire/III-Nitride hybrid structure. This technology uses both sides of a sapphire wafer to build device structures; on one side, making either Si or SiGe devices, and on the other side, making III-nitride device structures (e.g. GaN, InGaN, AlGaN). This innovation builds upon several previous innovations by NASA Langley Research Center, all relating to making silicon germanium semiconductor device structures on sapphire wafers.
The Technology
III-nitride devices are commonly made on sapphire substrates today for various commercial electronic and optoelectronic applications. Thus, this innovation relates directly to the combination of devices on opposite sides of the sapphire substrate. One possible device combination is to have LEDs one side and solar cells on the other, such as for displays.
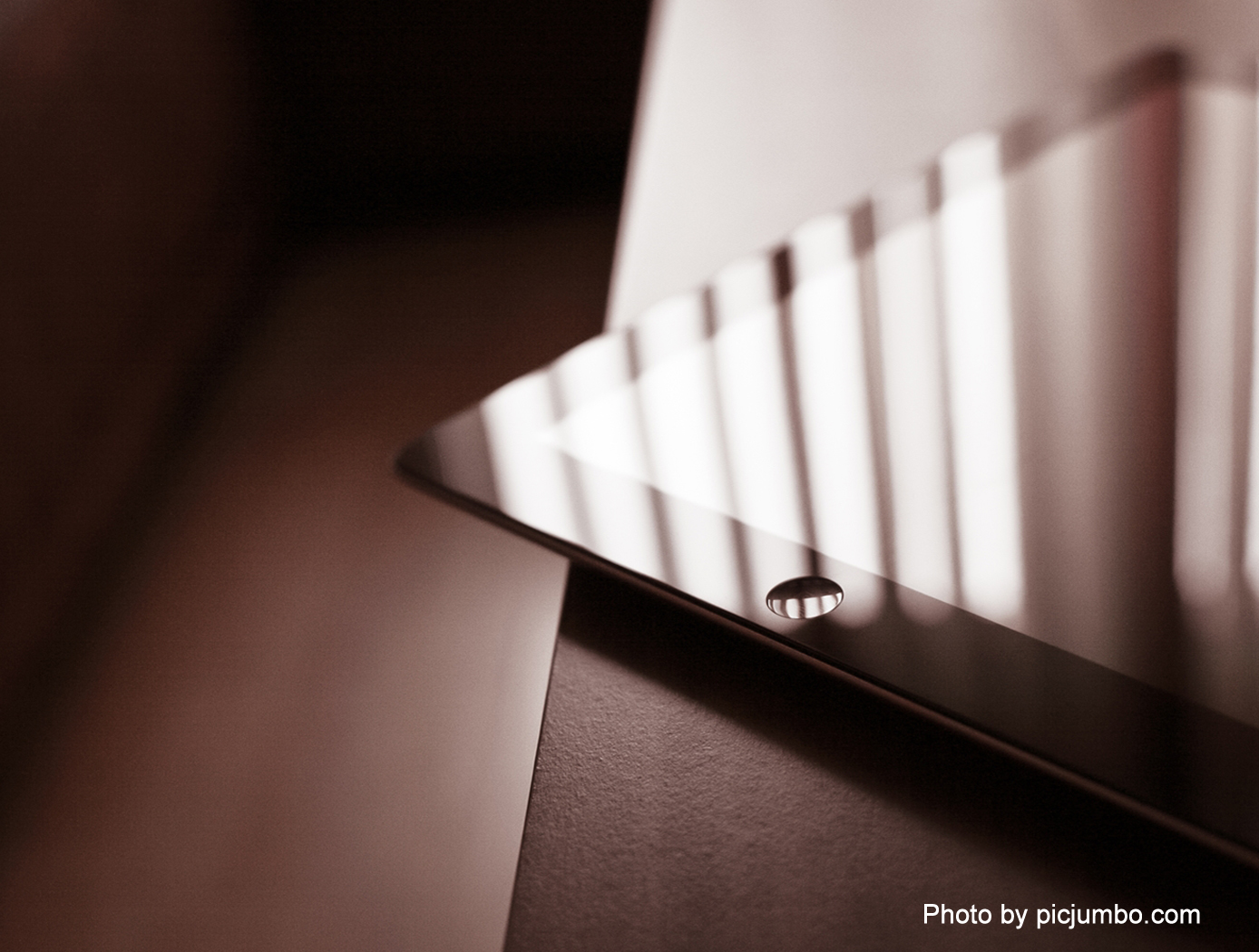
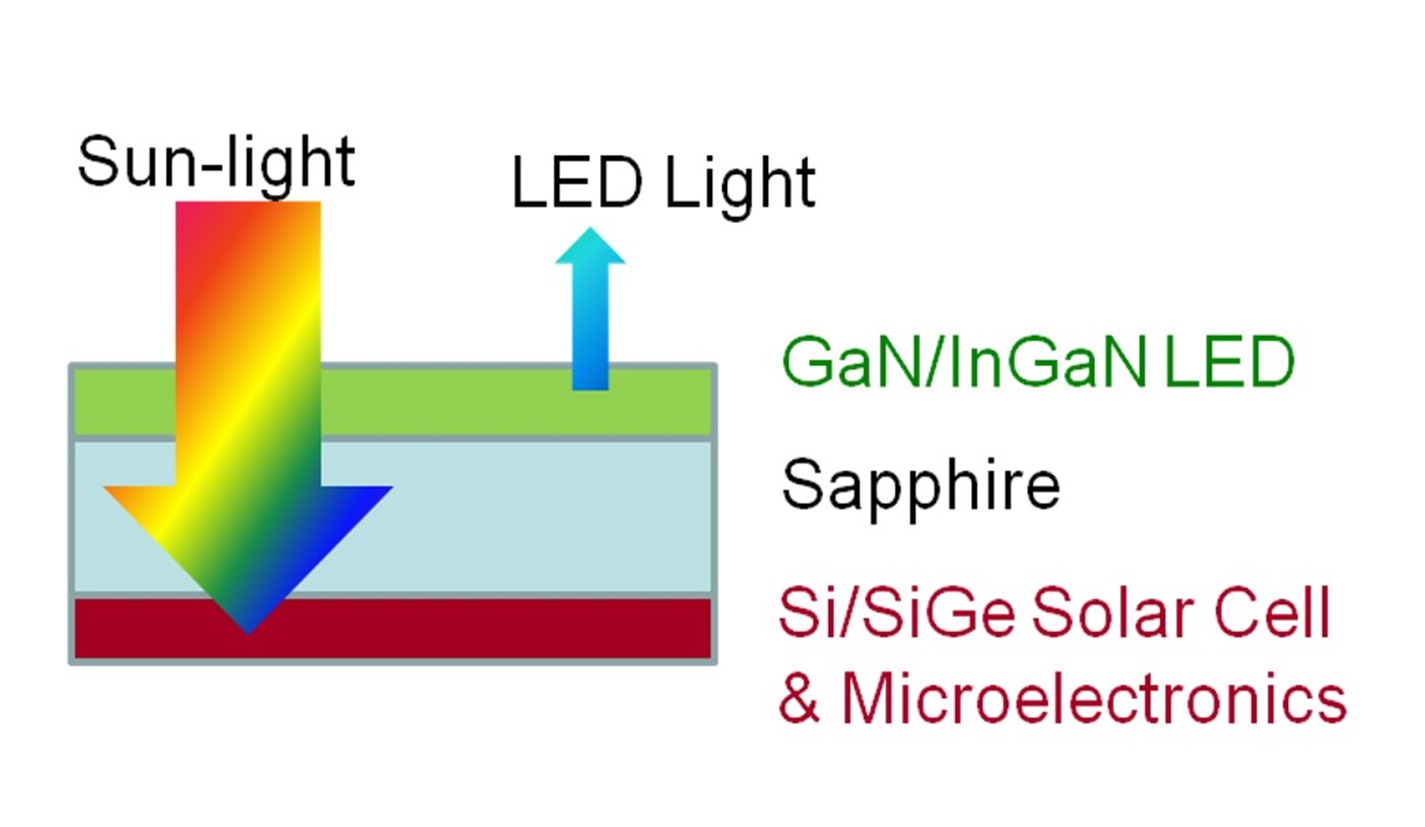
Benefits
- Novel semiconductor device structure that combines two distinctly different semiconductor materials, each with separate performance strengths, to create novel hybrid devices.
- Transparent substrate can provide back side illumination of solar cell.
- Builds upon proven technologies
Applications
- Self-powered display
- Solar cell-LED display
Similar Results

Epitaxy of SiGe and Other Compound Semiconductors
Several of the patented methods included in this suite of technologies enable super-hetero-epitaxy of rhombohedral/cubic compound semiconductors on specially oriented trigonal (e.g. sapphire) or hexagonal (e.g. quartz) crystal wafer substrates. This includes alignment of the growth crystal lattice with the underlying substrate lattice to minimize misfit strain-induced dislocation defects in the growing crystal. Thus thicker, defect-free crystal layers can be made. Rhombohedral/Cubic crystal twin defects which is 60 degree rotated on [111] orientation in a rhombohedral/cubic SiGe layer structure can be reduced to well less than 1% by volume, essentially providing a defect-free semiconductor material. Alternately, engineered lattice structures with a high degree of twinning can provide SiGe with improved thermoelectric properties due to the phonon scattering that inhibits thermal conduction without compromising electrical conductivity. Additional patented technologies in this suite provide for physical vapor deposition (PVD) growth methods utilizing molten sputtering targets and thermal control of heated substrates, including electron beam heating, in order to give the atoms in the sputtered vapor or on the substrate surface the energy needed for the desired crystal growth.
The remaining patented technologies enable x-ray diffraction methods for detecting and mapping crystal twin defects across the entire as-grown semiconductor layer. These defects are critical to the performance of any semiconductor device manufactured from such compound semiconductor materials.

Single Crystal SiGe/Sapphire Epitaxy
This innovation is based on a new fabrication method that alleviates the thermal loading requirement of the substrate, which previously required surface temperatures within the range of 850 to 900C. Our method employs a new thermal loading requirement of sapphire substrate for growing single crystal SiGe on sapphire substrate, in the range of 450 to 500C. SiGe/sapphire wafers produced via this process show a high reflectivity without the discoloration that appears in low quality films.

High Mobility Transport Layer Structures for Rhombohedral Si/Ge/SiGe Devices
Performance of solar cells and other electronic devices such as transistors can be improved greatly if carrier mobility is increased. Si and Ge have Type-II bandgap alignment in cubically strained and relaxed layers. Quantum well and super lattice with Si, Ge, and SiGe have been good noble structures to build high electron mobility layer and high hole mobility layers. However, the atomic lattice constant of Ge is bigger than that of Si and direct epitaxial growth generates large density of misfit dislocations which decrease carrier mobility and shorten device life time. So it required special buffer layers such as super lattice or gradient indexed layers to grow Ge on Si wafers or Si on Ge wafers. The growth of these buffer layers takes extra effort and time such as post-annealing process to remove dislocations by dislocation gliding inside buffer layer.
This invention is a fabrication method for high mobility layer structures of rhombohedrally aligned SiGe on a trigonal substrate. The invention utilizes C-plane (0001) Sapphire which has a triangle plane, and a Si (Ge) (C) (111) crystal or an alloy of group TV semiconductor (111) crystal grown on the Sapphire.

Integrated Multi-Color Light Emitting Device Made With Hybrid Crystal Structure
This technology is an integrated hybrid crystal LED display device that can emit red, green, and blue colors on one single wafer. Todays LEDs are built with many compound semiconductors with type-I direct bandgap energies of two different crystal structures. While Red, Orange, Yellow, Yellowish Green LEDs are commonly made with III-V semiconductor alloys of Aluminum Gallium Indium Phosphide (AlGaInP) and Aluminum Gallium Indium Arsenide (AlGaInAs) with cubic zinc blende crystal structures, the higher energy colors such as green, blue, purple, and Ultra-Violet(UV) LEDs are made with III-Nitride compound semiconductor of AlGaInN alloys with hexagonal wurtzite crystal structures. Because the atomic crystal structures are different for red LED and green/blue LEDs, the integration of these semiconductor LEDs as individual R, G, B pixels on one wafer was almost impossible or very difficult so far.

Reduced PIC fabrication cost by one-step method
The new fabrication method is an electron lithography scheme enabling monolithic integration of multiple photonic devices on a single PIC. The technology was demonstrated by integrating both a widely-tunable distributed Bragg reflector (DBR) and distributed feedback (DFB) lasers on the same substrate. By controlling the central gap width and etch depth along the laser mirror length (shown in the figure below) the reflectivities can be tuned and the desired laser characteristics can be achieved without additional lithography cycles.
Initially demonstrated on an indium phosphide substrate with DBR and DFB elements, the platform technology shows promise for various other materials and devices like III-V and II-VI semiconductors, silicon-on-insulator (SOI), and planar lightwave circuits (PLCs). With this versatility, the invention described here can streamline PIC production across diverse applications.
Proof-of-concept results showcase the lithographic technique’s ability to produce high-performance photonic devices with side-mode suppression ratios over 50 dB (figure on the right) and output powers exceeding 5 mW. These metrics, combined with the lithographic simplicity, highlight the technology’s potential to reduce costs and accelerate PIC manufacturing. Please note that NASA does not manufacture products itself for commercial scale.