Search
PATENT PORTFOLIO
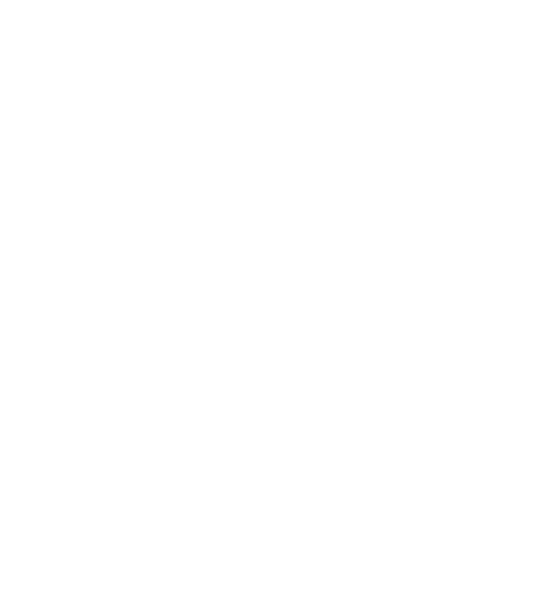
Aerospace
NASA has a wide range of advanced aerospace technologies that can be useful for both small companies seeking to introduce new solutions and large corporations looking to improve their capabilities. These technologies have the potential to transform the aerospace industry and drive the development of innovative solutions.
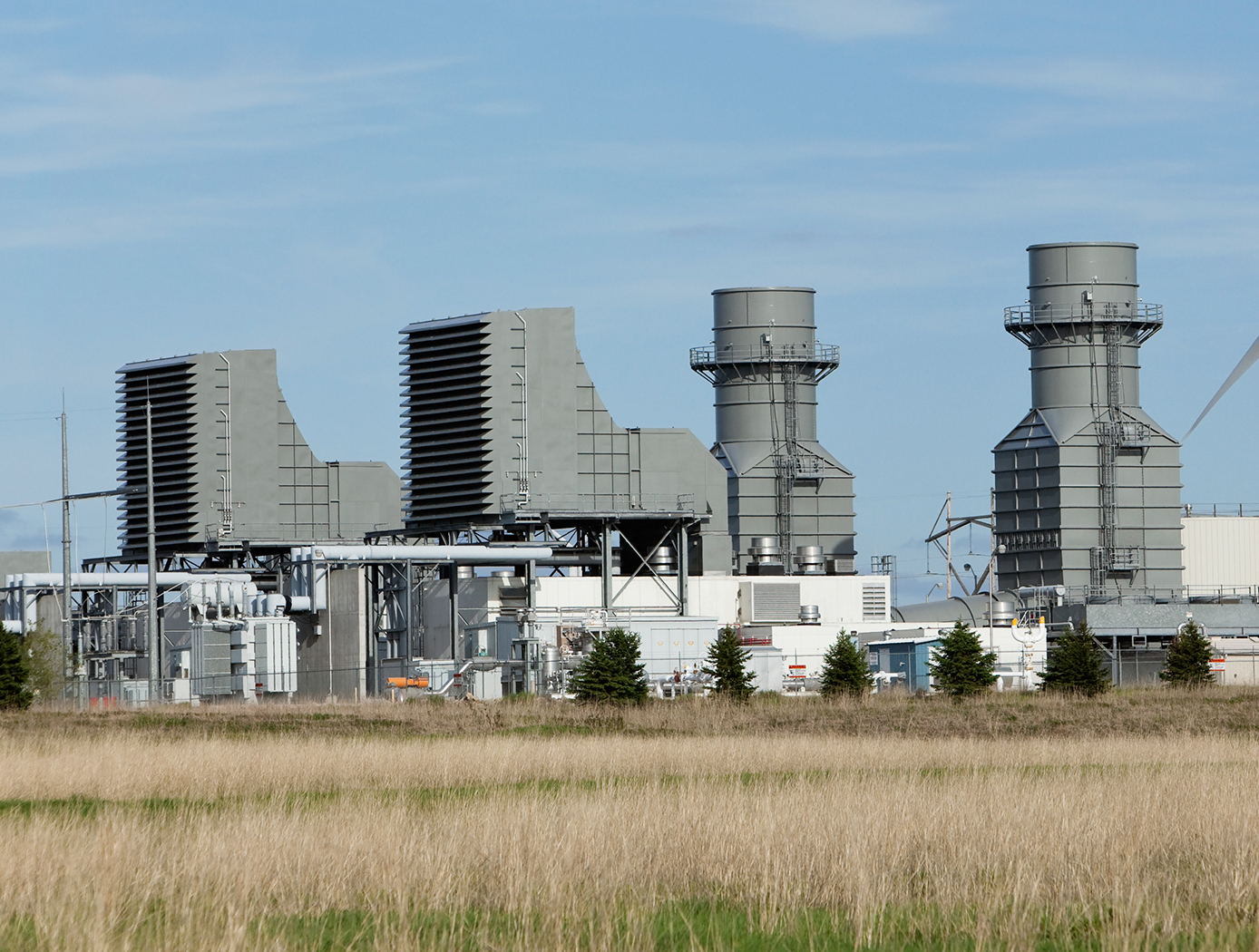
New Concepts in Film Cooling for Turbine Blades
In one of NASA Glenn's innovations, a shaped recess can be formed on a surface associated with fluid flow. Often V-shaped, this shaped recess can be configured to create or induce fluid effects, temperature effects, or shedding effects. For example, the shaped recess can be paired (upstream or downstream) with a cooling channel. The configuration of the shaped recess can mitigate the lift-off or separation of the cooling jets that are produced by the cooling channels, thus keeping the cooling jets trained on turbine blades and enhancing the effectiveness of the film-cooling process. The second innovation produced to improve film cooling addresses problems that occur when high-blowing ratios, such as those that occur during transient operation, threaten to diminish cooling effectiveness by creating jet detachment. To keep the cooling jet attached to the turbine blade, and also to spread the jet in the spanwise direction, NASA Glenn inventors have successfully used cooling holes that reduce loss by blowing in the upstream direction. In addition, fences may be used upstream of the holes to bend the cooling flow back toward the downstream direction to further reduce mixing losses. These two innovations represent a significant leap forward in making film cooling for turbine blades, and therefore the operation of turbine engines, more efficient.
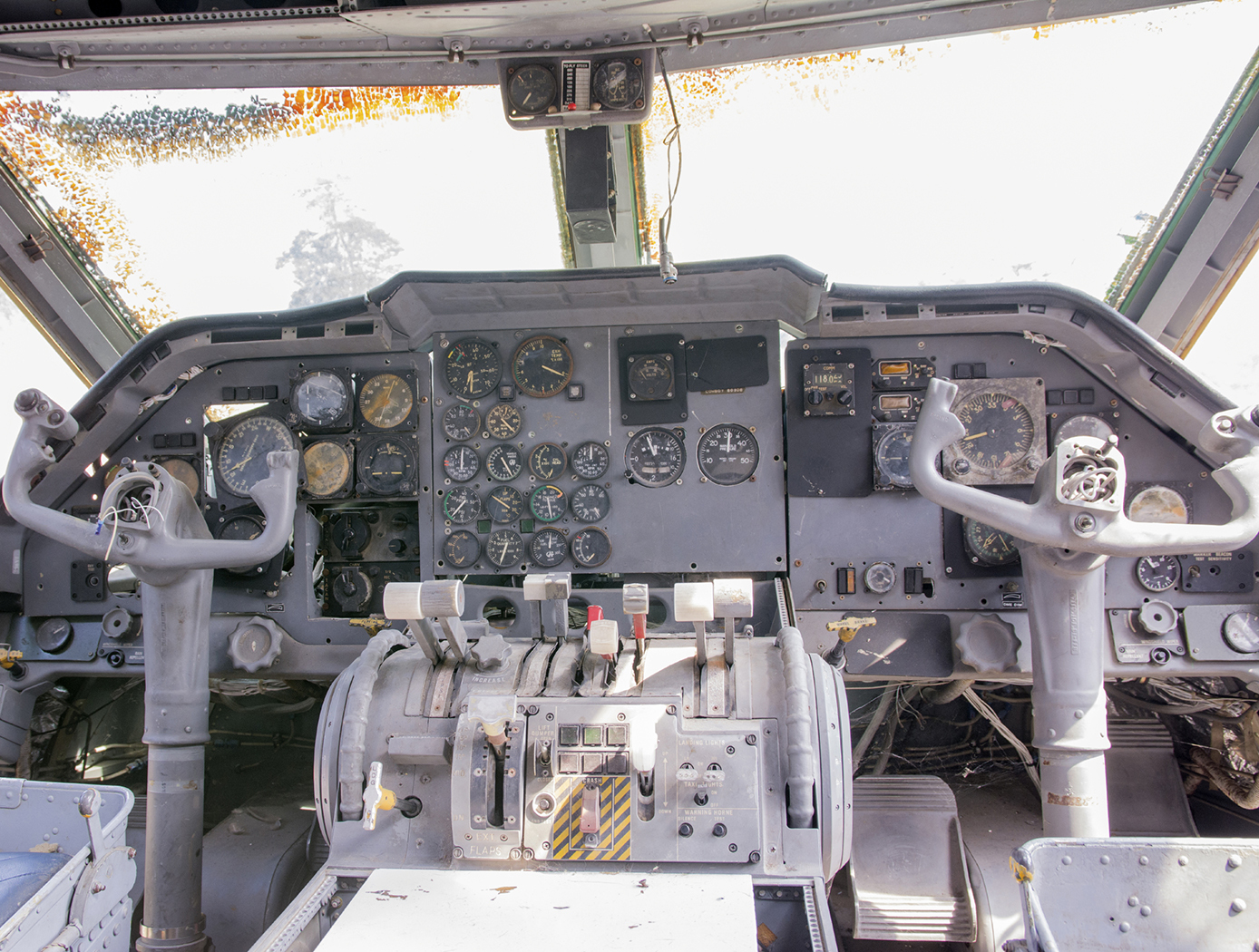
Conditionally Active Min-Max Limit Regulators
Current aircraft engine control logic uses a min-max control selection structure to prevent the engine from exceeding any safety or operational limits during transients due to throttle commands. This structure is inherently conservative and produces transient responses that are slower than necessary. By activating the NASA Glenn's conditionally active limit regulators, engine response can be improved while preserving all necessary safety limits. An engine controller using CA limit regulators will get a faster engine response while ensuring engine safety. The improved performance is attained by eliminating unnecessary limit regulator activations and by utilizing more of the available safety margins.
This is an early-stage technology requiring additional development. Glenn welcomes co-development opportunities.
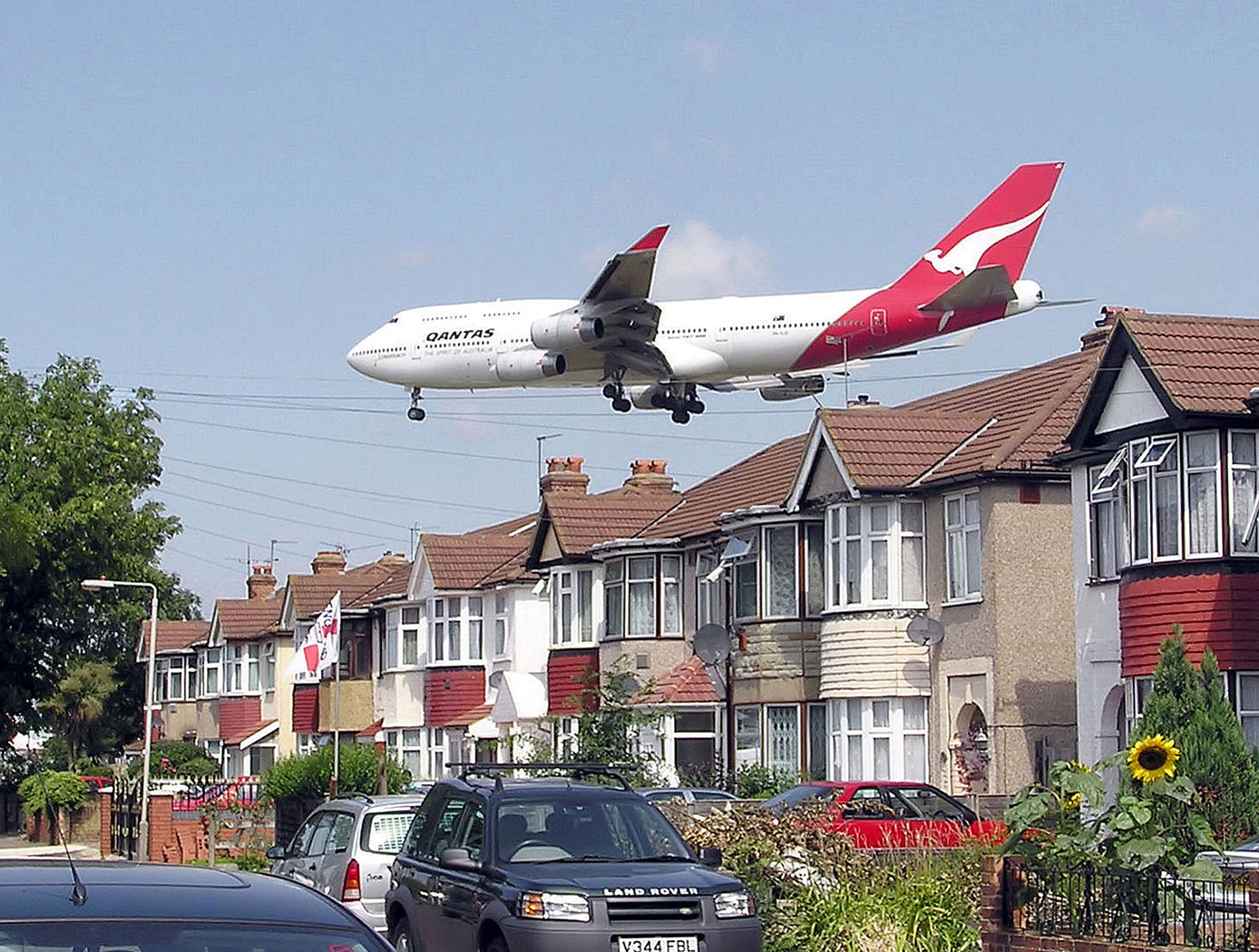
Compact, Lightweight, CMC-Based Acoustic Liner
NASA researchers are extending an existing oxide/oxide CMC sandwich structure concept that provides mono-tonal noise reduction. That oxide/oxide CMC has a density of about 2.8 g/cc versus the 8.4 g/cc density of a metallic liner made of IN625, thus offering the potential for component weight reduction. The composites have good high-temperature strength and oxidation resistance, allowing them to perform as core liners at temperatures up to 1000°C (1832°F). NASA's innovation uses cells of different lengths or effective lengths within a compact CMC-based liner to achieve broadband noise reduction. NASA has been able to optimize the performance of the proposed acoustic liner by using improved design tools that help reduce noise over a specified frequency range. One such improvement stems from the enhanced understanding of variable-depth liners, including the benefits of alternate channel shapes/designs (curved, bent, etc.). These new designs have opened the door for CMC-based acoustic liners to offer core engine noise reduction in a lighter, more compact package. As a first step toward demonstrating advanced concepts, an oxide/oxide CMC acoustic testing article with different channel lengths was tested. Bulk absorbers could also be used, either in conjunction with or in place of the liners internal chambers, to reduce noise further if desired.
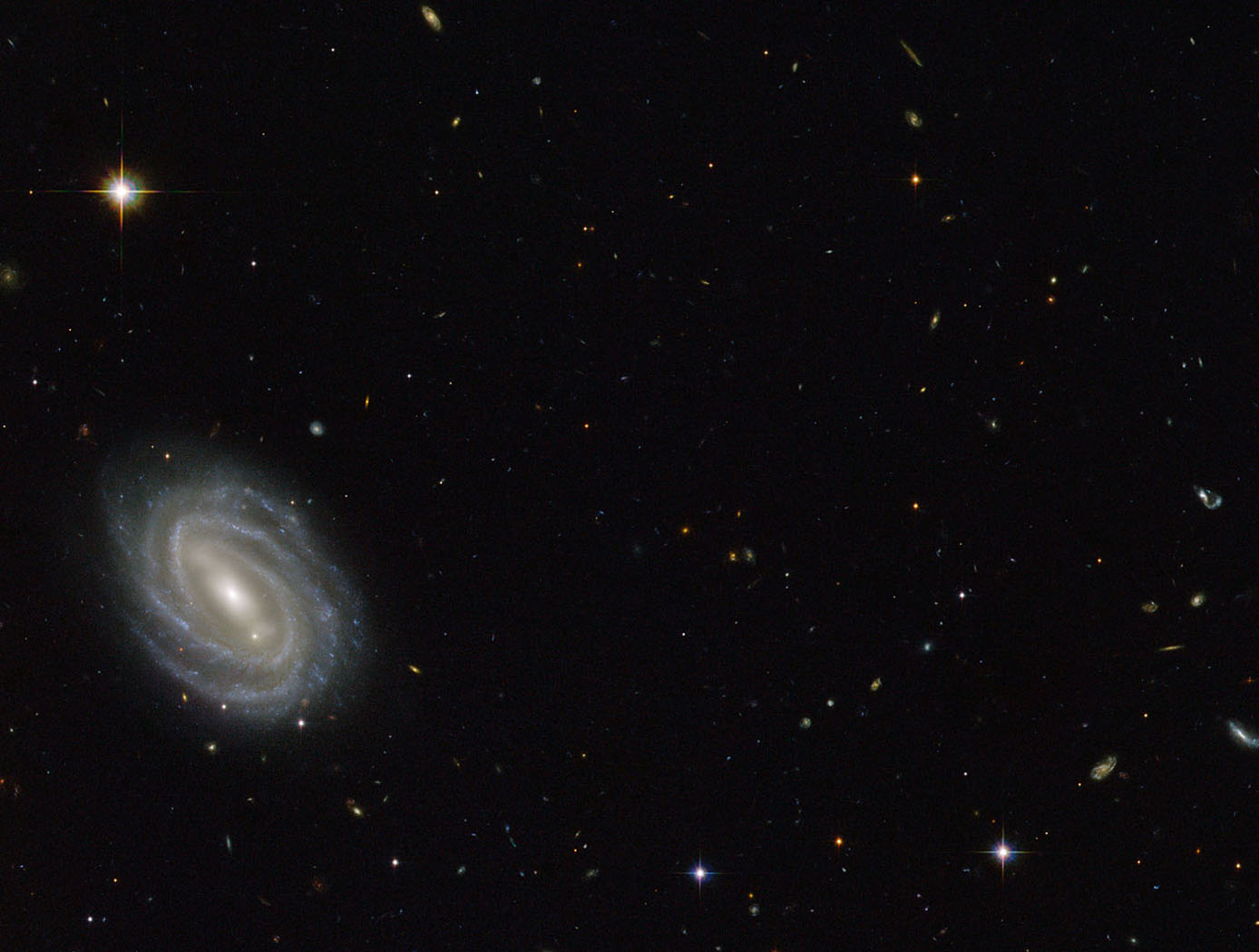
SpaceCube Demonstration Platform
The HST SM4 SpaceCube flight spare was modified to create an experiment called the SpaceCube Demonstration Platform (SC DP) for use on the MISSE7 Space Station payload (in collaboration with NRL). It is designed to serve as an on-orbit platform for demonstrating advanced fault tolerance technologies.
With the use of Xilinx commercial Virtex4 FX60 FPGAs, the fault tolerant framework allows the system to recover from radiation upsets that occur in the rad-soft parts (Virtex4 FPGA logic, embedded PPCs in Virtex4 FPGAs, SDRAM and Flash), the C&DH system that runs simultaneously on both Virtex4 FPGAs that uses a robust telemetry packet structure, checksums, and the rad-hard service FPGA to validate incoming telemetry. The ability to be reconfigured from the ground while in orbit is a novel benefit, as well as is the onboard compression capabilities that allow compressed files from the ground to be uploaded to the SpaceCube.
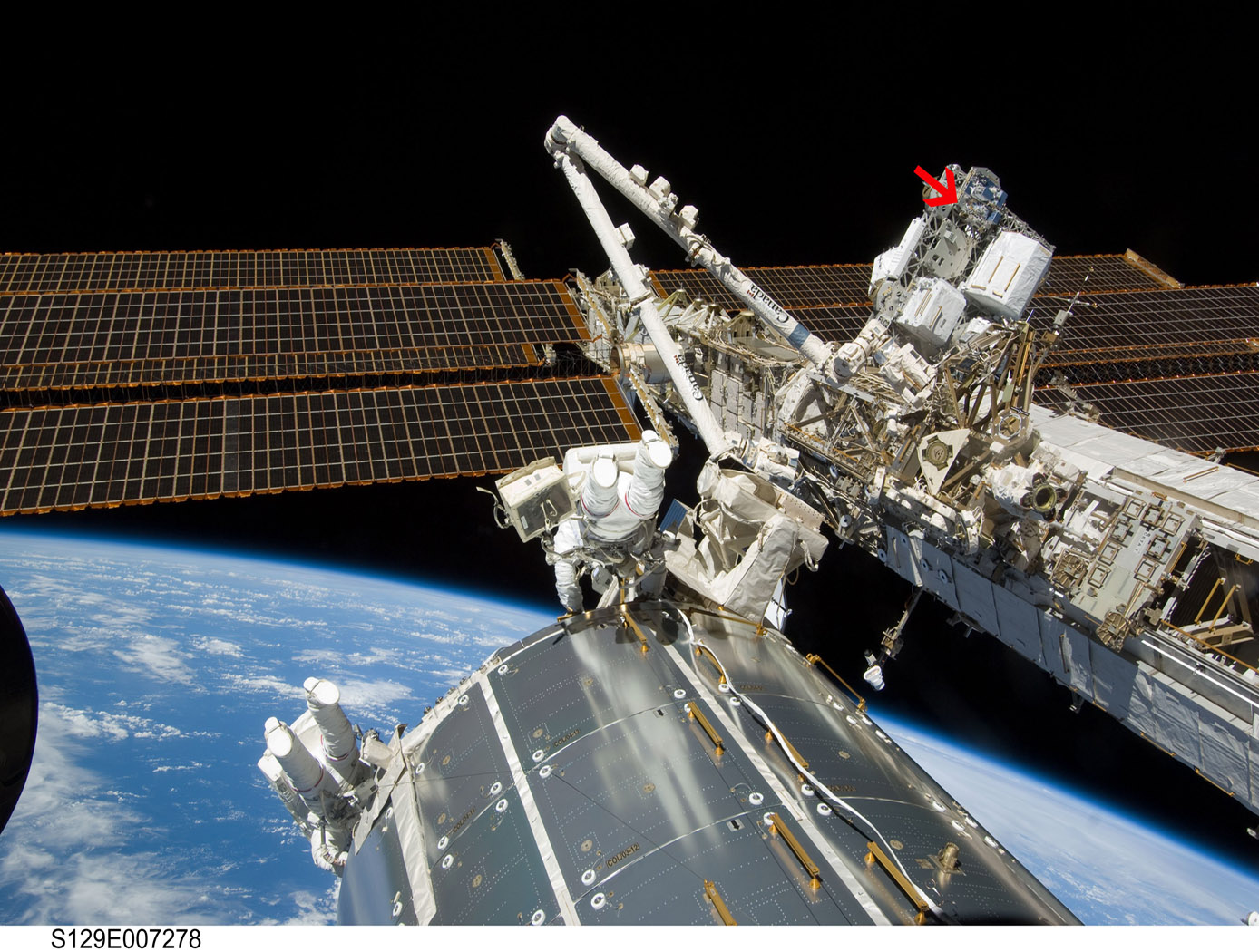
Novel Antenna Concept for CubeSat Platforms
The side walls and railing rods of a CubeSat are replaced by RF radiators that double as supporting structures. The RF radiators are hollow railing rods with inner dimensions that function as a waveguide to carry RF energy at a desired frequency. Radiating slots are cut on two of the four sides of hollow tubes tube that are open to outside environment. Different operating frequency antennas may be placed at each of the Cubesats four corners. Thus the railing rods provide RF antenna functionality in addition to structurally supporting the CubeSat structure.
While this technology was designed for Cubesats, it may be utilized in any technology that utilizes a structural frame. The advantages of this system are increased reliability due to the elimination of deployment mechanisms and decreased payloads. Higher frequency
antennas with increased gain and directivity may be embedded into the rails. These higher frequencies are especially useful for remote sensing.
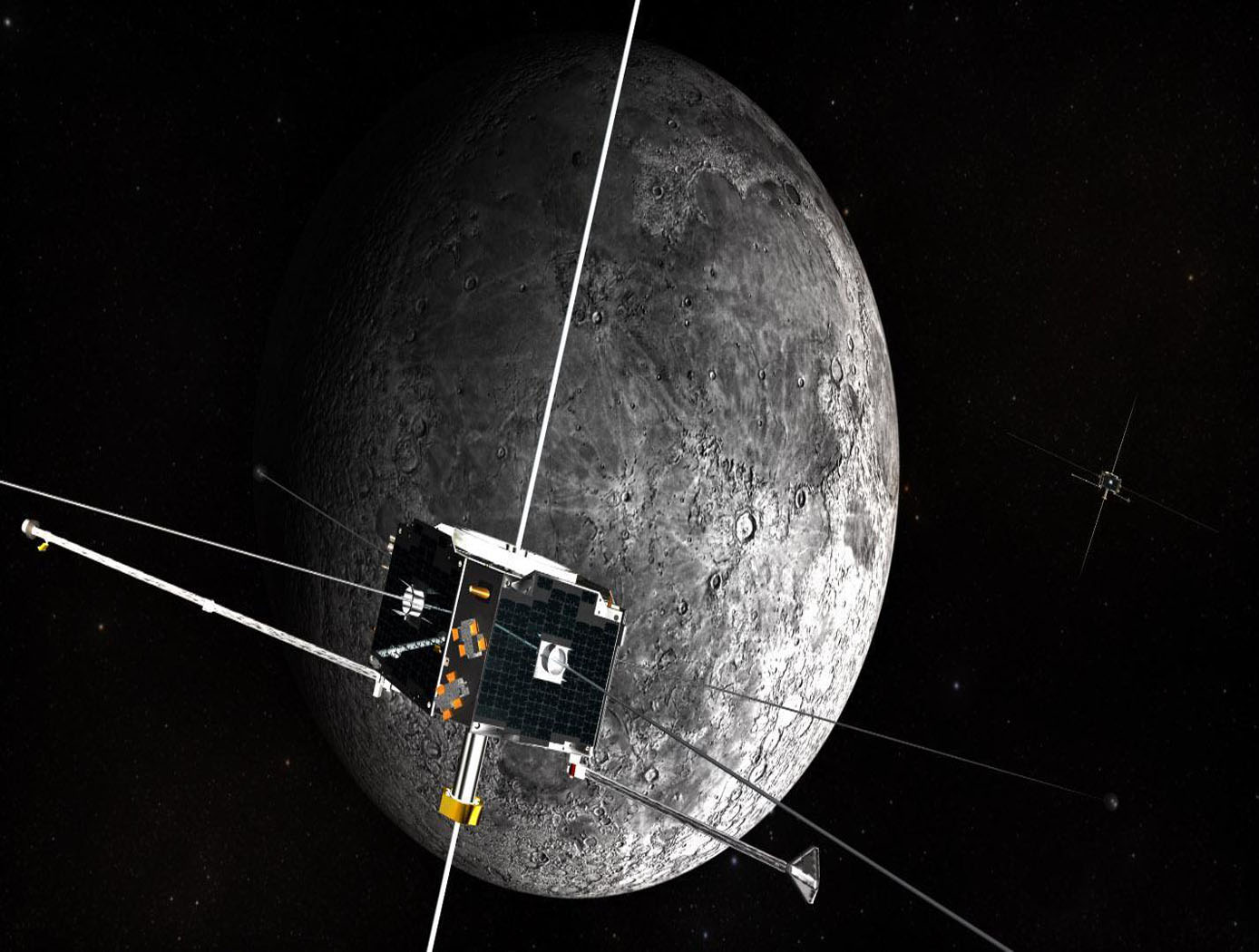
Method for Transferring a Spacecraft from Geosynchronous Transfer Orbit to Lunar Orbit
The invention presents a trajectory design whereby a spacecraft can be launched as a secondary payload into a Geosynchronous Transfer Orbit (GTO) and through a series of maneuvers to reach lunar orbit. The trajectory analysis begins by identifying acceptable ranges of lunar orbit altitude and inclination values. The unique features of this method includes the use of either a leading or trailing edge lunar flyby to achieve an orbit inclination in the lunar orbit plane from a GTO launched at any time of day. This technique is applicable to secondary spacecraft that share a ride to space resulting in a substantially reduced cost, and with no control of the launch conditions. Major advantages of this design include the relatively short (maximum) lunar transfer duration (<3 months, less than half of that required for a Sun-Earth weak-stability boundary transfer), simplicity and consistency of design (again compared to a Sun-Earth weak stability boundary transfer).

Improved Ground Collision Avoidance System
This critical safety tool can be used for a wider variety of aircraft, including general aviation, helicopters, and unmanned aerial vehicles (UAVs) while also improving performance in the fighter aircraft currently using this type of system.
Demonstrations/Testing
This improved approach to ground collision avoidance has been demonstrated on both small UAVs and a Cirrus SR22 while running the technology on a mobile device. These tests were performed to the prove feasibility of the app-based implementation of this technology. The testing also characterized the flight dynamics of the avoidance maneuvers for each platform, evaluated collision avoidance protection, and analyzed nuisance potential (i.e., the tendency to issue false warnings when the pilot does not consider ground impact to be imminent).
Armstrong's Work Toward an Automated Collision Avoidance System
Controlled flight into terrain (CFIT) remains a leading cause of fatalities in aviation, resulting in roughly 100 deaths each year in the United States alone. Although warning systems have virtually eliminated CFIT for large commercial air carriers, the problem still remains for fighter aircraft, helicopters, and GAA.
Innovations developed at NASAs Armstrong Flight Research Center are laying the foundation for a collision avoidance system that would automatically take control of an aircraft that is in danger of crashing into the ground and fly it—and the people inside—to safety. The technology relies on a navigation system to position the aircraft over a digital terrain elevation data base, algorithms to determine the potential and imminence of a collision, and an autopilot to avoid the potential collision. The system is designed not only to provide nuisance-free warnings to the pilot but also to take over when a pilot is disoriented or unable to control the aircraft.
The payoff from implementing the system, designed to operate with minimal modifications on a variety of aircraft, including military jets, UAVs, and GAA, could be billions of dollars and hundreds of lives and aircraft saved. Furthermore, the technology has the potential to be applied beyond aviation and could be adapted for use in any vehicle that has to avoid a collision threat, including aerospace satellites, automobiles, scientific research vehicles, and marine charting systems.
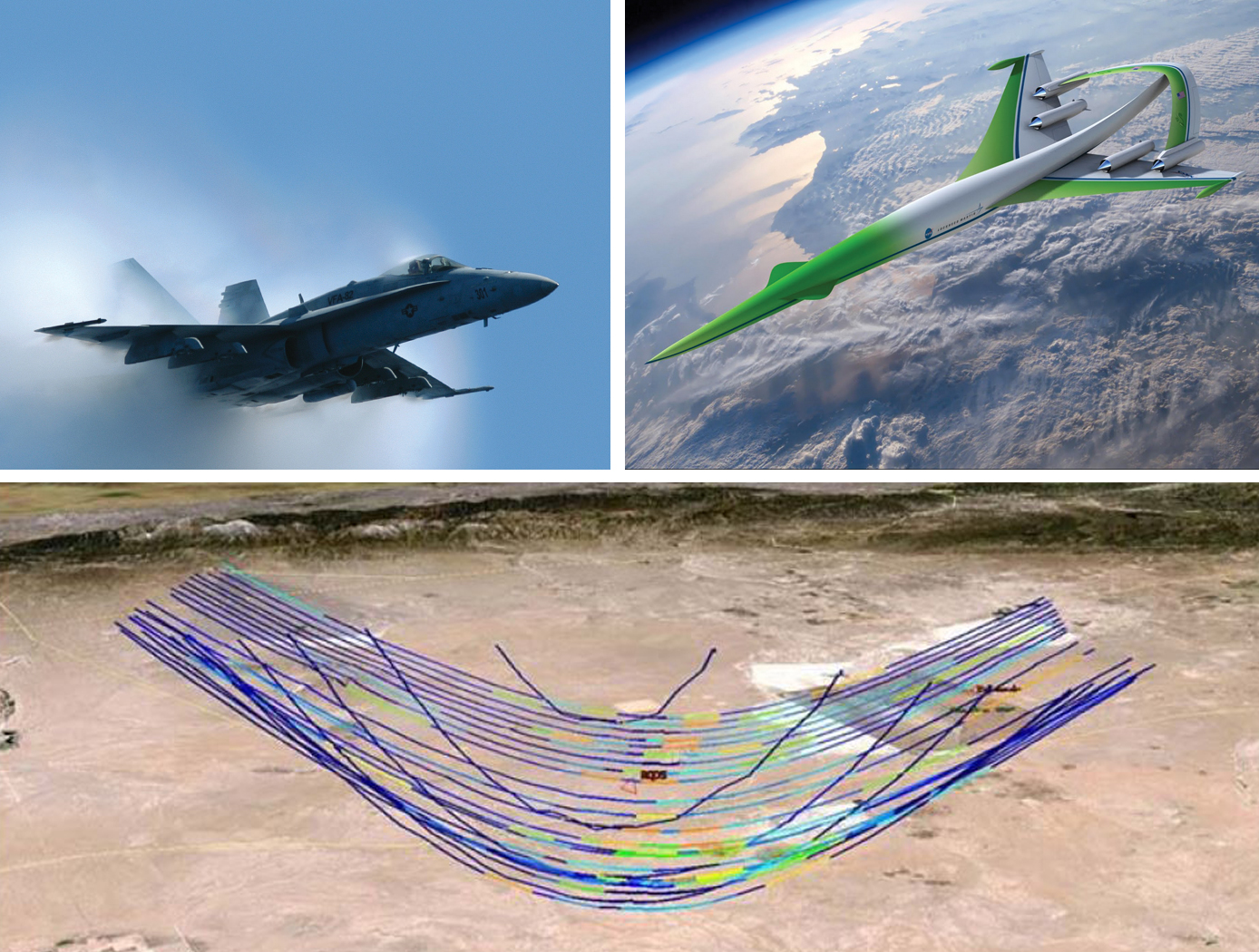
Interactive Sonic Boom Display
A supersonic shock wave forms a cone of pressurized air molecules that propagates outward in all directions and extends to the ground. Factors that influence sonic booms include aircraft weight, size, and shape, in addition to its altitude, speed, acceleration and flight path, and weather or atmospheric conditions. NASA's Real-Time Sonic Boom Display takes all these factors into account and enables pilots to control and mitigate sonic boom impacts.
How It Works
Armstrong's technology incorporates 3-dimensional (3D) Earth modeling and inputs of 3D atmospheric data. Central to the innovation is a processor that calculates significant information related to the potential for sonic booms based on an aircraft's specific operation. The processor calculates the sonic boom near a field source based on aircraft flight parameters, then ray traces the sonic boom to a ground location taking into account the near field source, environmental condition data, terrain data, and aircraft information. The processor signature ages the ray trace information to obtain a ground boom footprint and also calculates the ray trace information to obtain Mach cutoff condition altitudes and airspeeds.
Prediction data are integrated with a real-time, local-area moving-map display that is capable of displaying the aircraft's currently generated sonic boom footprint at all times. A pilot can choose from a menu of pre-programmed maneuvers such as accelerations, turns, or pushovers and the predicted sonic boom footprint for that maneuver appears on the map display. This allows pilots to select or modify a flight path or parameters to either avoid generating a sonic boom or to place the sonic boom in a specific location. The system also provides pilots with guidance on how to execute a chosen maneuver.
Why It Is Better
No other system exists to manage sonic booms in-flight. NASA's approach is unique in its ability to display in real time the location and intensity of shock waves caused by supersonic aircraft. The system allows pilots to make in-flight adjustments to control the intensity and location of sonic booms via an interactive display that can be integrated into cockpits or flight control rooms. The technology has been in use in Armstrong control rooms and simulators since 2000 and has aided several sonic boom research projects.
Aerospace companies have the technological capability to build faster aircraft for overland travel; however, the industry has not yet developed a system to support flight planning and management of sonic booms. The Real-Time Sonic Boom Display fills this need. The capabilities of this cutting-edge technology will help pave the way toward overland supersonic flight, as it is the key to ensuring that speed increases can be accomplished without disturbing population centers.
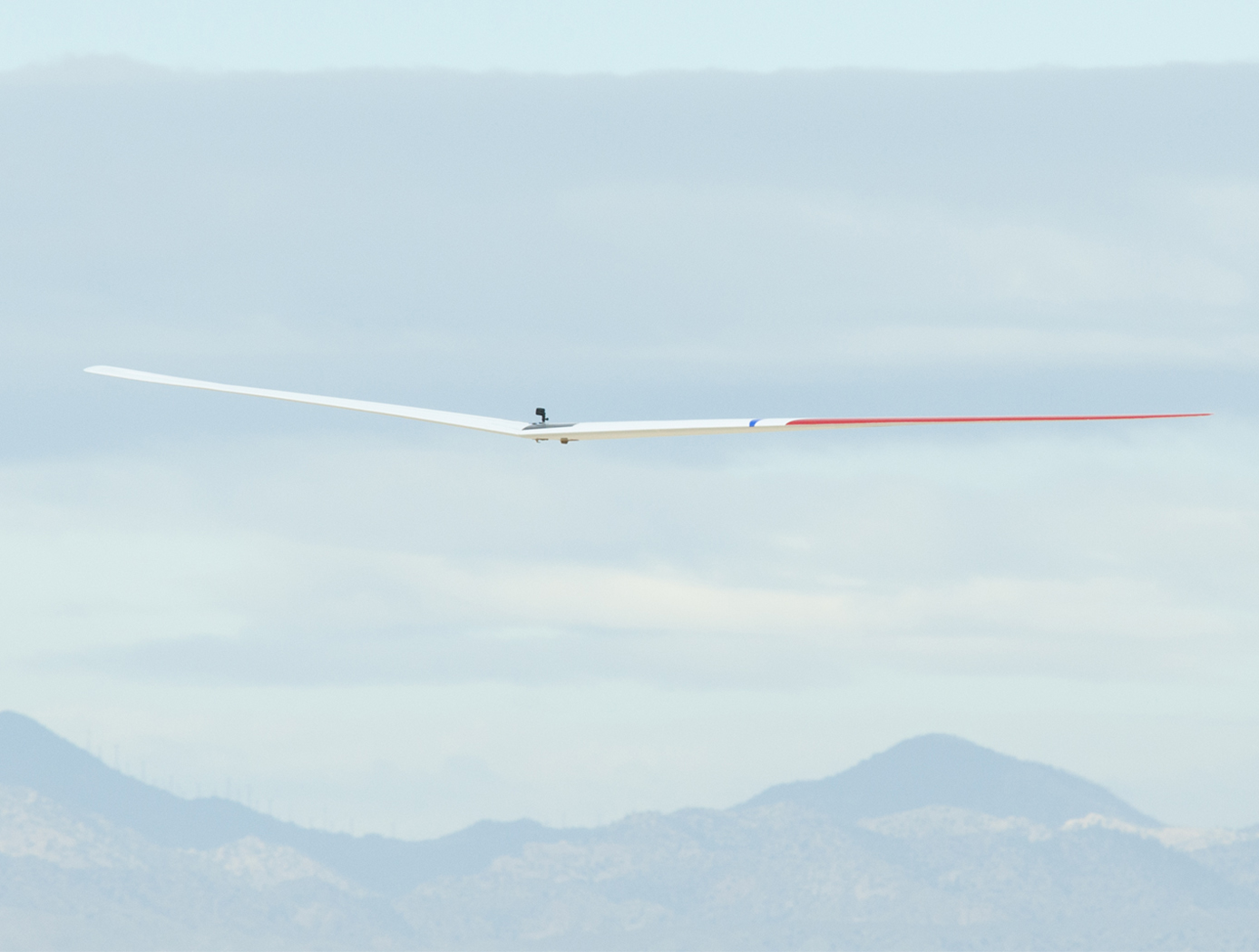
New Wing Design Exponentially Increases Total Aircraft Efficiency
Adverse yaw, present in current aircraft design, is the adverse horizontal movement around a vertical axis of an aircraft; the yaw opposes the direction of a turn. As an aircraft turns, differential drag of the left and right wings while banking contributes to aircraft yaw. Proverse yaw—yawing in the same direction as a turn—would optimize aircraft performance. Initial results from flight experiments at Armstrong demonstrated that this wing design unequivocally established proverse yaw. This wing design further reduces drag due to lift at the same time.
How It Works
The Armstrong team (supported by a large contingent of NASA Aeronautics Academy interns) built upon the 1933 research of the German engineer Ludwig Prandtl to design and validate a scale model of a non-elliptical loaded wing that reduces drag and increases efficiency.
The key to the innovation is reducing the drag of the wing through use of an alternative bell-shaped spanload, as opposed to the conventional elliptical spanload. To achieve the bell spanload, designers used a sharply tapered wing, with 12 percent less wing area than the comparable elliptical spanload wing. The new wing has 22 percent more span and 11 percent less area, resulting in an immediate 12 percent drag reduction.
Furthermore, using twist to achieve the bell spanload produces induced thrust at the wing tips, and this forward thrust increases when lift is increased at the wingtips for roll control. The result is that the aircraft rolls and yaws in the same direction as a turn, eliminating the need for a vertical tail. When combined with a blended-wing body, this approach maximizes aerodynamic performance, minimizes weight, and optimizes flight control.
Why It Is Better
Conventional aircraft make use of elliptical loaded wings to minimize drag. However, achieving aircraft stability and control in conventional elliptical wings produces a strong adverse yaw component in roll control (i.e., the aircraft will yaw the opposite direction with application of roll control). Therefore, a vertical tail or some other method of direct yaw control is required, such as split elevons for use as drag rudders. The use of elliptical wings also results in a suboptimal amount of structure to carry the integrated wing bending moment.
Adopting the bell-shaped spanload change results in an immediate 12 percent drag reduction. In addition, optimization of the overall aircraft configuration is projected to achieve additional significant overall performance increases.
View more patents