Search
aerospace
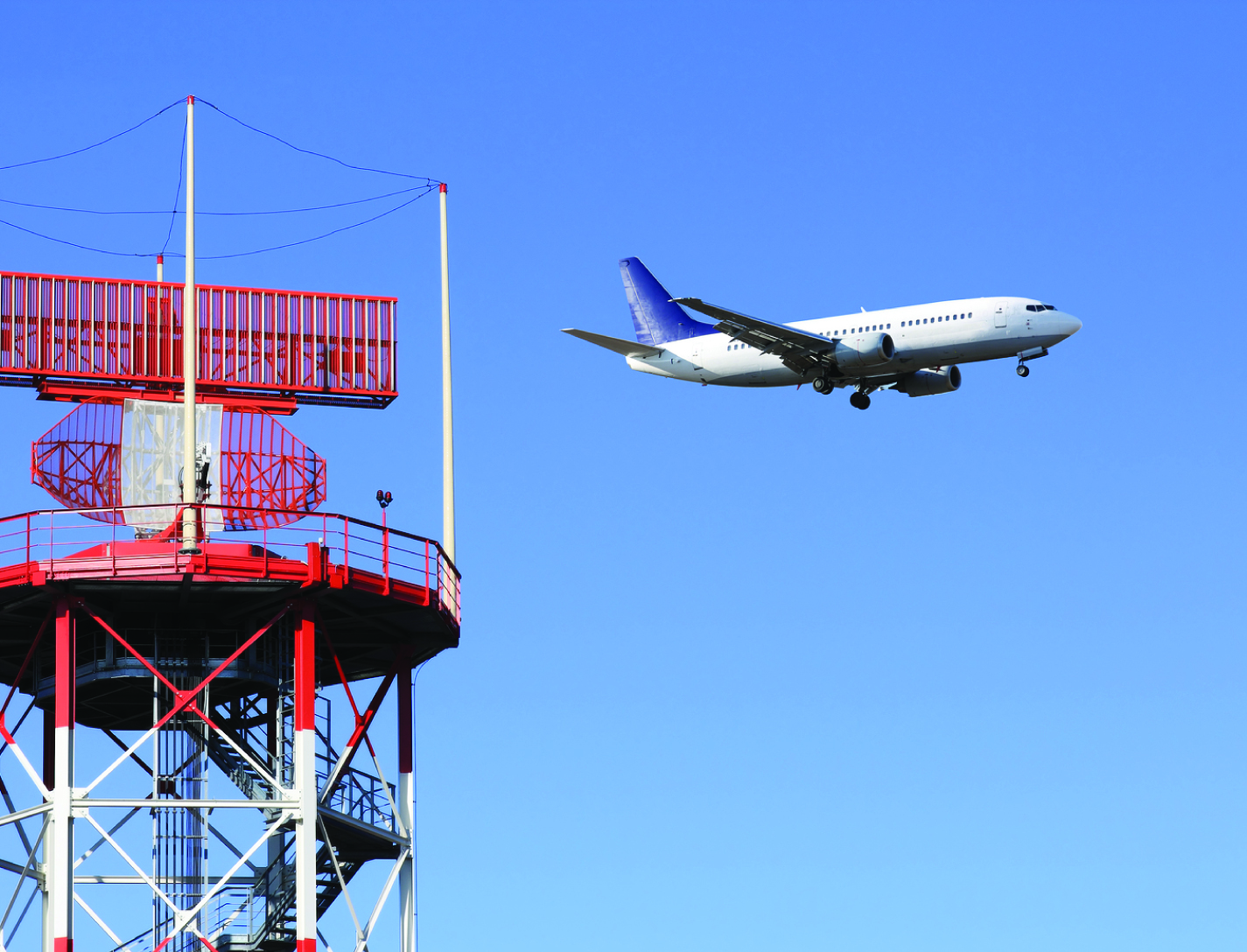
Dynamic Weather Routes Tool
Every 12 seconds, the Dynamic Weather Route (DWR) automation system computes and analyzes trajectories for en-route flights. DWR first identifies flights that could save 5 or more flying minutes (wind-corrected) by flying direct to a downstream return fix on their current flight plan. Eligible return fixes are limited so as not to take flights too far off their current route or interfere with arrival routings near the destination airport. Using the direct route as a reference route, DWR inserts up to two auxiliary waypoints as needed to find a minimum-delay reroute that avoids the weather and returns the flight to its planned route at the downstream fix. If a reroute is found that can save 5 minutes or more relative to the current flight plan, the flight is posted to a list displayed to the airline or FAA user. Auxiliary waypoints are defined using fix-radial-distance format, and a snap to nearby named fix option is available for todays voice-based communications. Users may also adjust the alert criteria, nominally set to 5 minutes, based on their workload and desired potential savings for their flights. A graphical user interface enables visualization of proposed routes on a traffic display and modification, if necessary, using point, click, and drag inputs. If needed, users can adjust the reroute parameters including the downstream return fix, any inserted auxiliary waypoints, and the maneuver start point. Reroute metrics, including flying time savings (or delay) relative to the current flight plan, proximity to current and forecast weather, downstream sector congestion, traffic conflicts, and conflicts with special use airspace are all updated dynamically as the user modifies a proposed route.
electrical and electronics
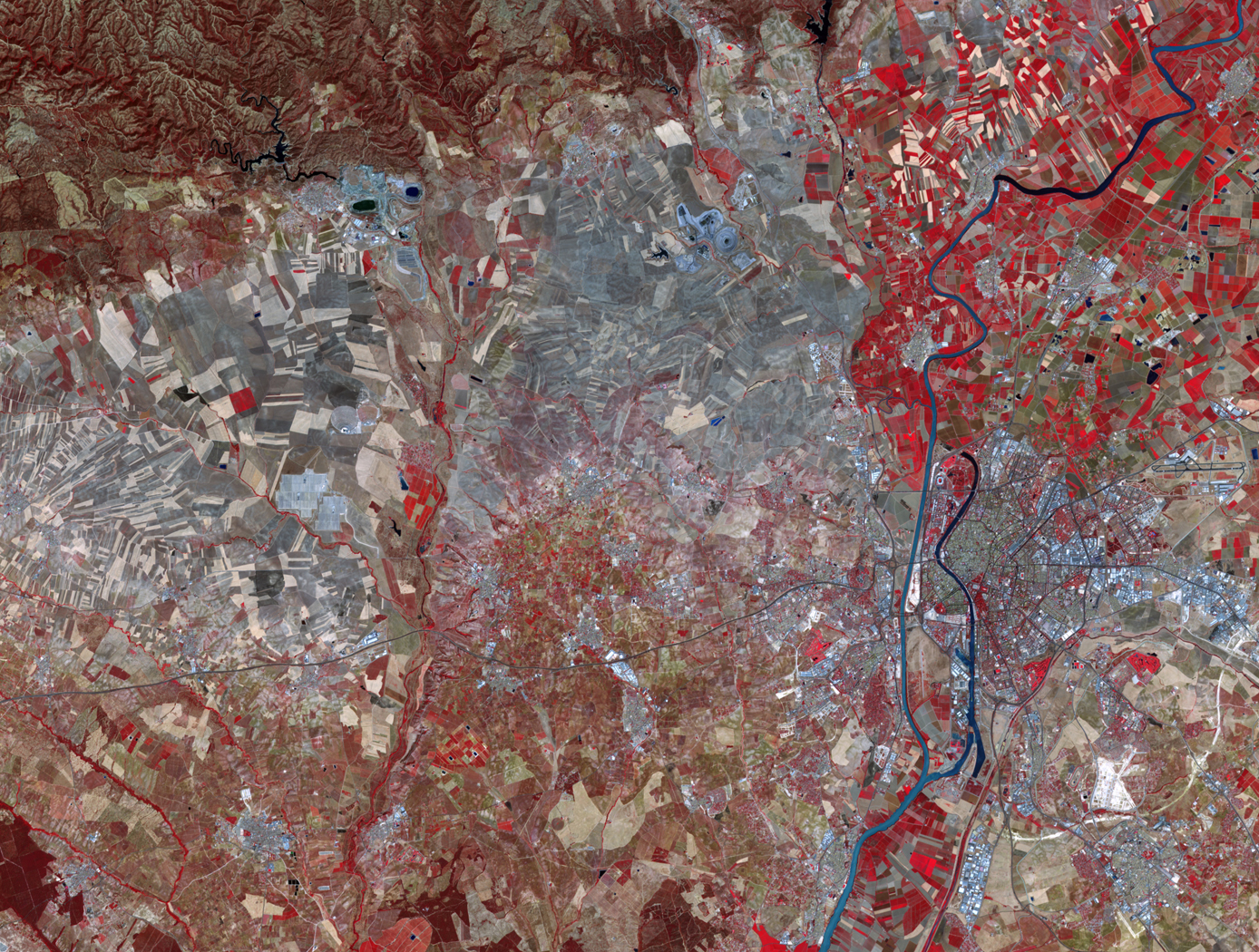
Using the Power Grid for Geophysical Imaging
This technology utilizes the U.S. high-voltage power transmission grid system as an extremely large antenna to extract unprecedented spatiotemporal space physical and geological information from distributed GIC observations. GICs are measured using differential a magnetometer technique involving one fluxgate magnetometer under the transmission line and another reference magnetometer station nearby. The reference station allows subtraction of the natural field from the line measurement, leaving only the GIC-related Biot-Savart field. This allows inversion of the GIC amplitude. The magnetometer stations are designed to operate autonomously. They are low-cost, enabling large scale application with a large number of measurement locations.
communications
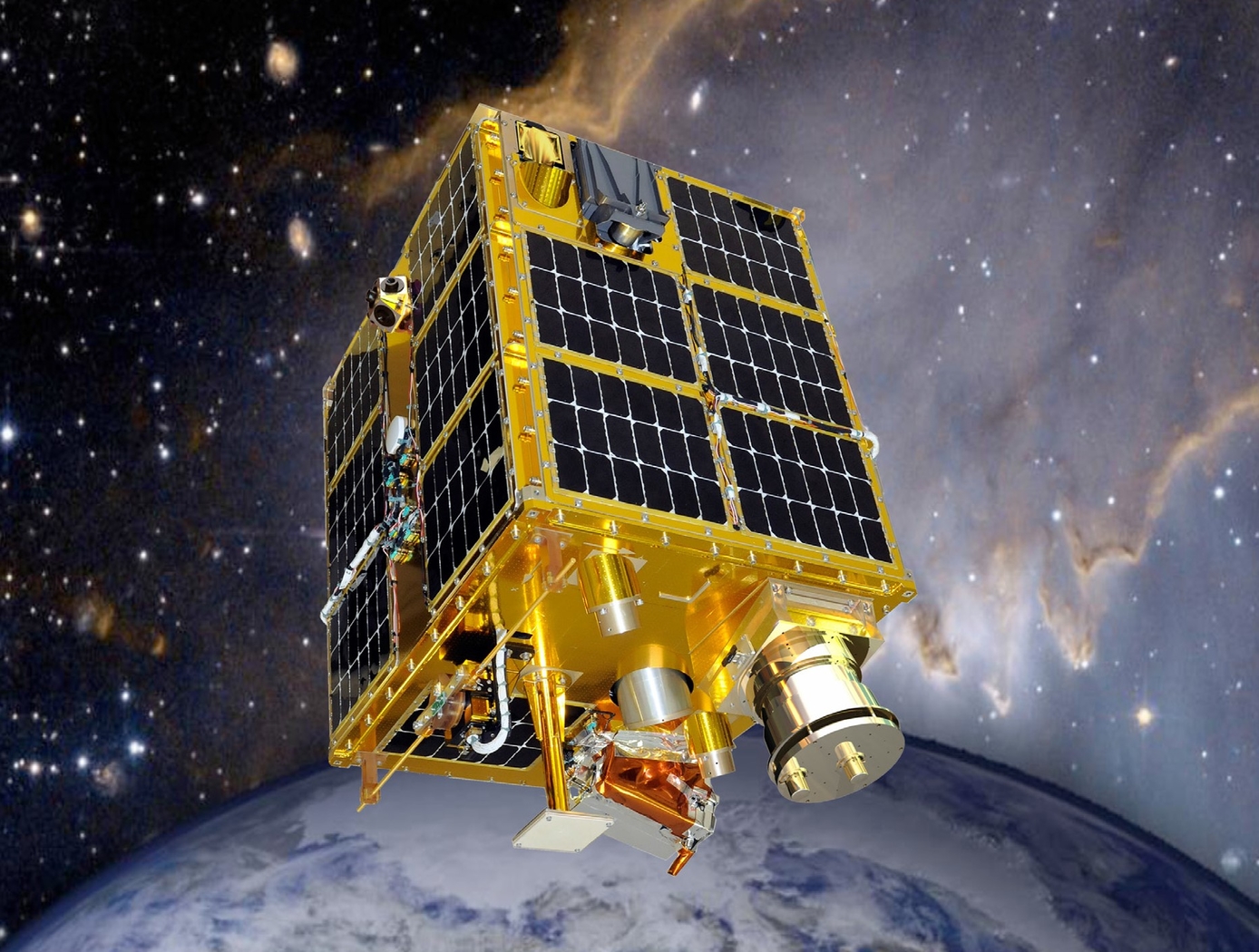
High-Speed, Low-Cost Telemetry Access from Space
NASA's SDR uses Field-Programmable Gate Array (FPGA) technology to enable flexible performance on orbit. A first-generation FM-modulated transceiver is capable of operating at up to 1 Mbps downlink and 50 kbps uplink, full duplex. An FPGA performs Reed-Solomon (255,223) encoding, decoding, and bit synchronization, providing Consultative Committee for Space Data Systems (CCSDS) and Near Earth Network (NEN) telemetry protocol compatibility. The transceiver accepts data from the onboard flight computer via a source synchronous RS422 interface.
NASA's second-generation full duplex SDR, known as PULSAR (programmable ultra-lightweight system-adaptable radio, Figures 1 and 2 below) incorporates command receiver and telemetry transmitters, as well as updated processing and power capabilities. An S-band command receiver offers a max uplink data rate of 300 Kbps and built-in QPSK demodulation. X- and S-Band telemetry transmitters offer a max downlink data rate of 150 Mbps and flexible forward-error correction (FEC) using Reed-Solomon encoding (LDPC rate 7/8 and 1/2 convolution in development), and it uses QPSK modulation. The use of FEC adds an order of magnitude increase in telemetry throughput due to an improved coding gain. An onboard FPGA uses high-speed logic for uplink/downlink and encoding/decoding processes. Balloon flight testing has been conducted and is ongoing for PULSAR.
optics

Video Acuity Measurement System
The Video Acuity metric is designed to provide a unique and meaningful measurement of the quality of a video system. The automated system for measuring video acuity is based on a model of human letter recognition. The Video Acuity measurement system is comprised of a camera and associated optics and sensor, processing elements including digital compression, transmission over an electronic network, and an electronic display for viewing of the display by a human viewer. The quality of a video system impacts the ability of the human viewer to perform public safety tasks, such as reading of automobile license plates, recognition of faces, and recognition of handheld weapons. The Video Acuity metric can accurately measure the effects of sampling, blur, noise, quantization, compression, geometric distortion, and other effects. This is because it does not rely on any particular theoretical model of imaging, but simply measures the performance in a task that incorporates essential aspects of human use of video, notably recognition of patterns and objects. Because the metric is structurally identical to human visual acuity, the numbers that it yields have immediate and concrete meaning. Furthermore, they can be related to the human visual acuity needed to do the task. The Video Acuity measurement system uses different sets of optotypes and uses automated letter recognition to simulate the human observer.
communications
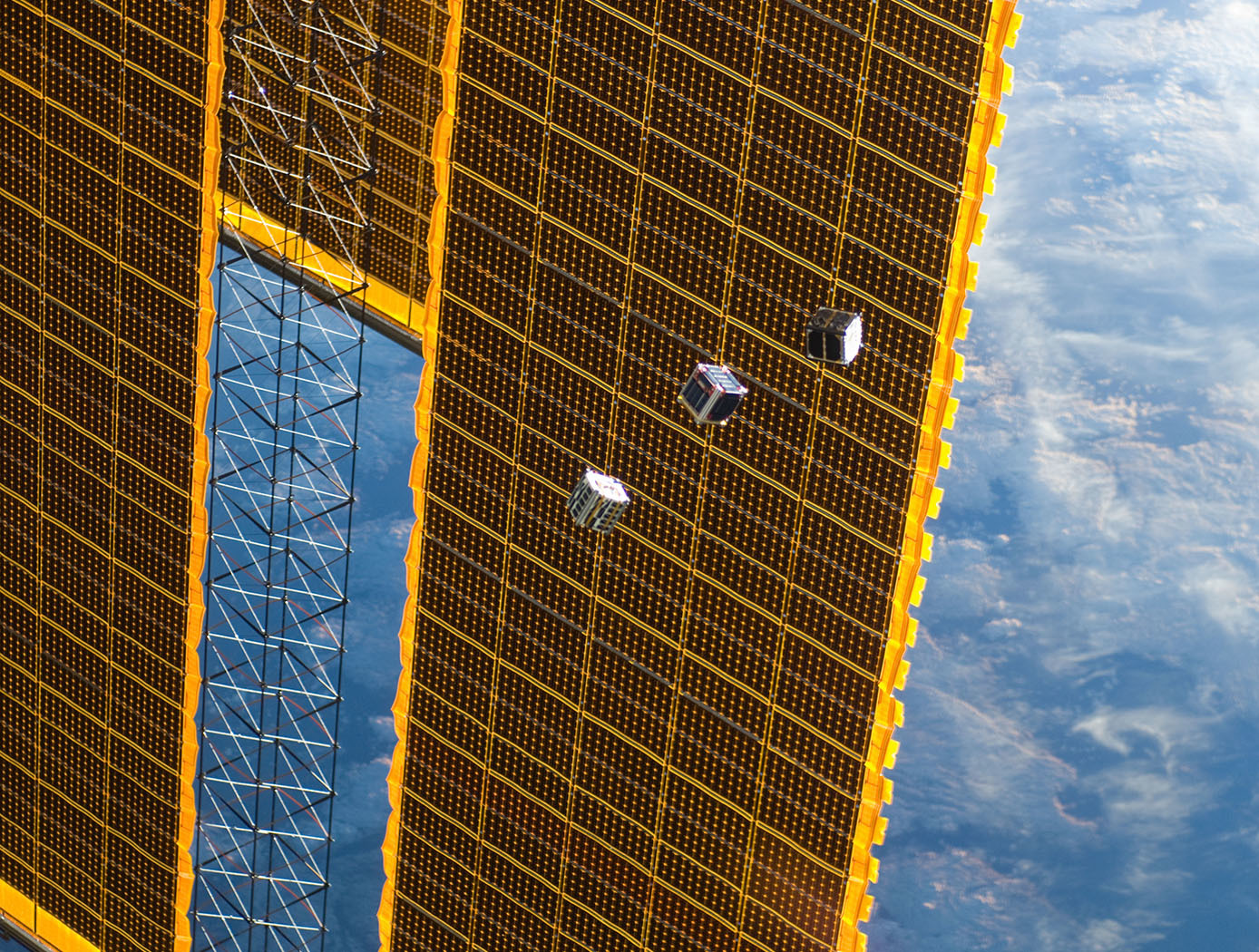
Tunable Multi-Tone, Multi-Band, High-Frequency Synthesizer
Glenn's revolutionary new multi-tone, high-frequency synthesizer can enable a major upgrade in the design of high data rate, wide-band satellite communications links, in addition to the study of atmospheric effects. Conventional single-frequency beacon transmitters have a major limitation: they must assume that atmospheric attenuation and group delay effects are constant at all frequencies across the band of interest. Glenn's synthesizer overcomes this limitation by enabling measurements to be made at multiple frequencies across the entire multi-GHz wide frequency, providing much more accurate and actionable readings.
This novel synthesizer consists of a solid-state frequency comb or harmonic generator that uses step-recovery semiconductor diodes to generate a broad range of evenly spaced harmonic frequencies, which are coherent and tunable over a wide frequency range. These harmonics are then filtered by a tunable bandpass filter and amplified to the necessary power level by a tunable millimeter-wave power amplifier. Next, the amplified signals are transmitted as beacon signals from a satellite to a ground receiving station. By measuring the relative signal strength and phase at ground sites the atmospheric induced effects can be determined, enabling scientists to gather essential climate data on hurricanes and climate change. In addition, the synthesizer can serve as a wideband source in place of a satellite transponder, making it easier to downlink high volumes of collected data to the scientific community. Glenn's synthesizer enables a beacon transmitter that, from the economical CubeSat platform, offers simultaneous, fast, and more accurate wideband transmission from space through the Earth's atmosphere than has ever been possible before.
Electrical and Electronics
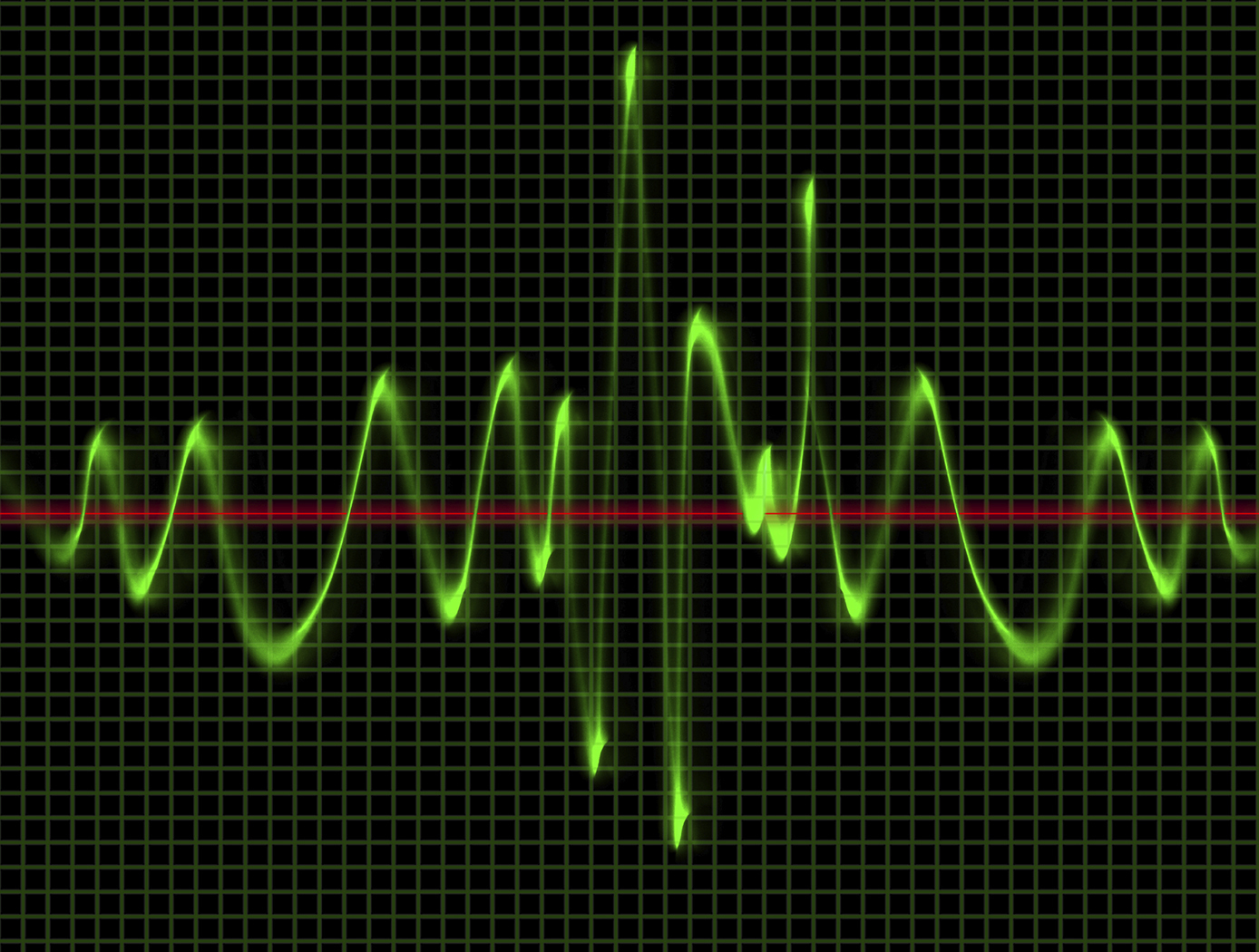
Dynamic Range Enhancement of High-Speed Data Acquisition Systems
Electronic waveforms exist that exceed the capabilities of state-of-the-art data acquisition hardware that is commonly available. The electronic waveforms that need to be measured simultaneously contain wide bandwidth, high frequency content, a DC reference, high dynamic range, and a high crest factor. The NASA Glenn high-speed data acquisition system creates a voltage compression effect with a custom transfer function that is adapted to the voltage range, frequency bandwidth, and electrical impedance of both the test article and data acquisition device. The compression transfer function is later reversed (or decompressed) with a software algorithm to restore the original signal's voltage from the acquired data. The data is thus improved via better signal-to-noise ratio, better low-amplitude accuracy, better resolution, and preservation of high-frequency spectral content. The circuit can be realized with either passive components or both active and passive components. Either realization is specialized for the test article and data acquisition hardware.
This is an early-stage technology requiring additional development. Glenn welcomes co-development opportunities.
information technology and software
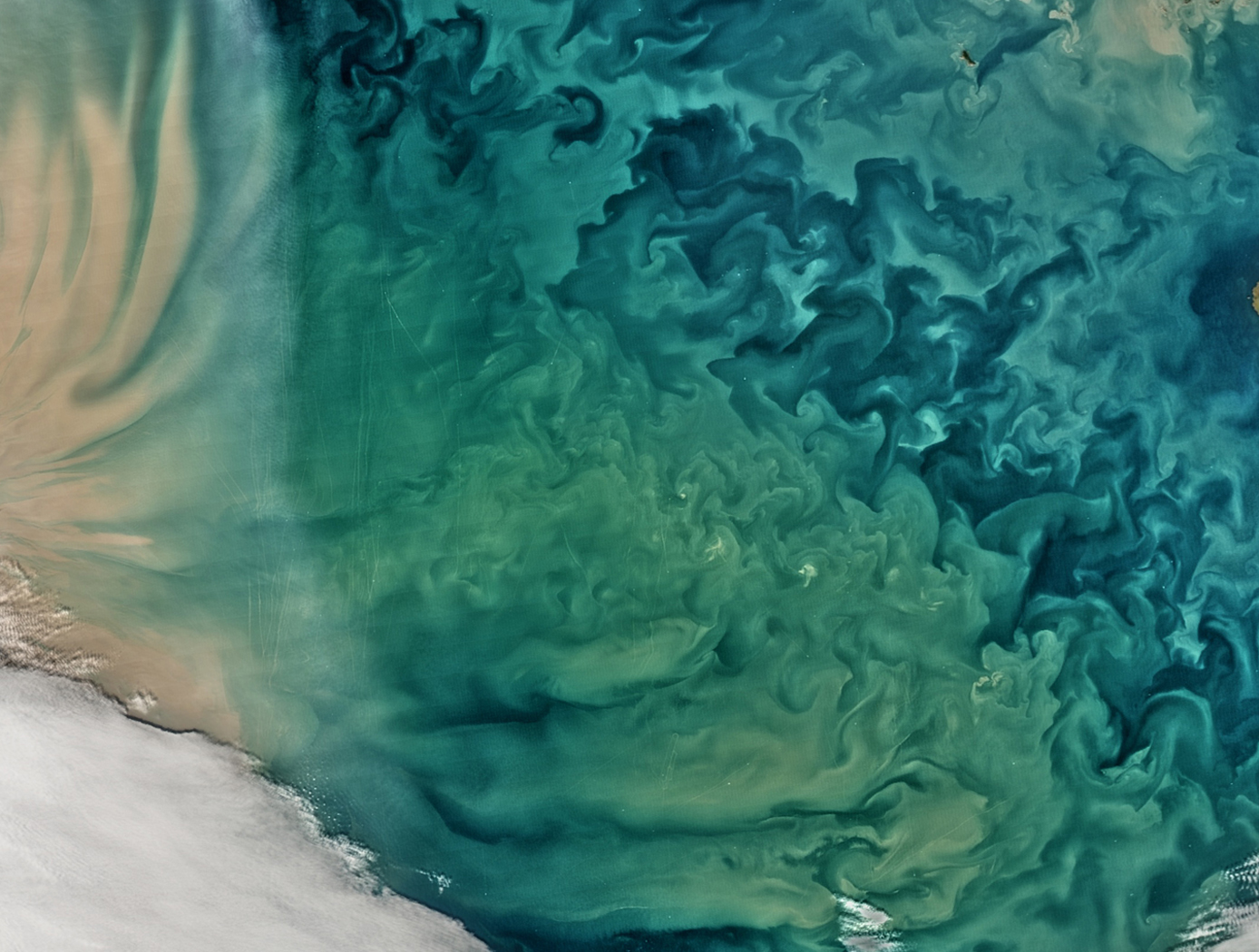
MERRA/AS and Climate Analytics-as-a-Service (CAaaS)
NASA Goddard Space Flight Center now offers a new capability for meeting this Big Data challenge: MERRA Analytic Services (MERRA/AS). MERRA/AS combines the power of high-performance computing, storage-side analytics, and web APIs to dramatically improve customer access to MERRA data. It represents NASAs first effort to provide Climate Analytics-as-a-Service.
Retrospective analyses (or reanalyses) such as MERRA have long been important to scientists doing climate change research. MERRA is produced by NASAs Global Modeling and Assimilation Office (GMAO), which is a component of the Earth Sciences Division in Goddards Sciences and Exploration Directorate. GMAOs research and development activities aim to maximize the impact of satellite observations in climate, weather, atmospheric, and land prediction using global models and data assimilation. These products are becoming increasingly important to application areas beyond traditional climate science.
MERRA/AS provides a new cloud-based approach to storing and accessing the MERRA dataset. By combining high-performance computing, MapReduce analytics, and NASAs Climate Data Services API (CDS API), MERRA/AS moves much of the work traditionally done on the client side to the server side, close to the data and close to large compute power. This reduces the need for large data transfers and provides a platform to support complex server-side data analysesit enables Climate Analytics-as-a-Service.
MERRA/AS currently implements a set of commonly used operations (such as avg, min, and max) over all the MERRA variables. Of particular interest to many applications is a core collection of about two dozen MERRA land variables (such as humidity, precipitation, evaporation, and temperature). Using the RESTful services of the Climate Data Services API, it is now easy to extract basic historical climatology information about places and time spans of interest anywhere in the world. Since the CDS API is extensible, the community can participate in MERRA/ASs development by contributing new and more complex analytics to the MERRA/AS service.
MERRA/AS demonstrates the power of CAaaS and advances NASAs ability to connect data, science, computational resources, and expertise to the many customers and applications it serves.
Sensors
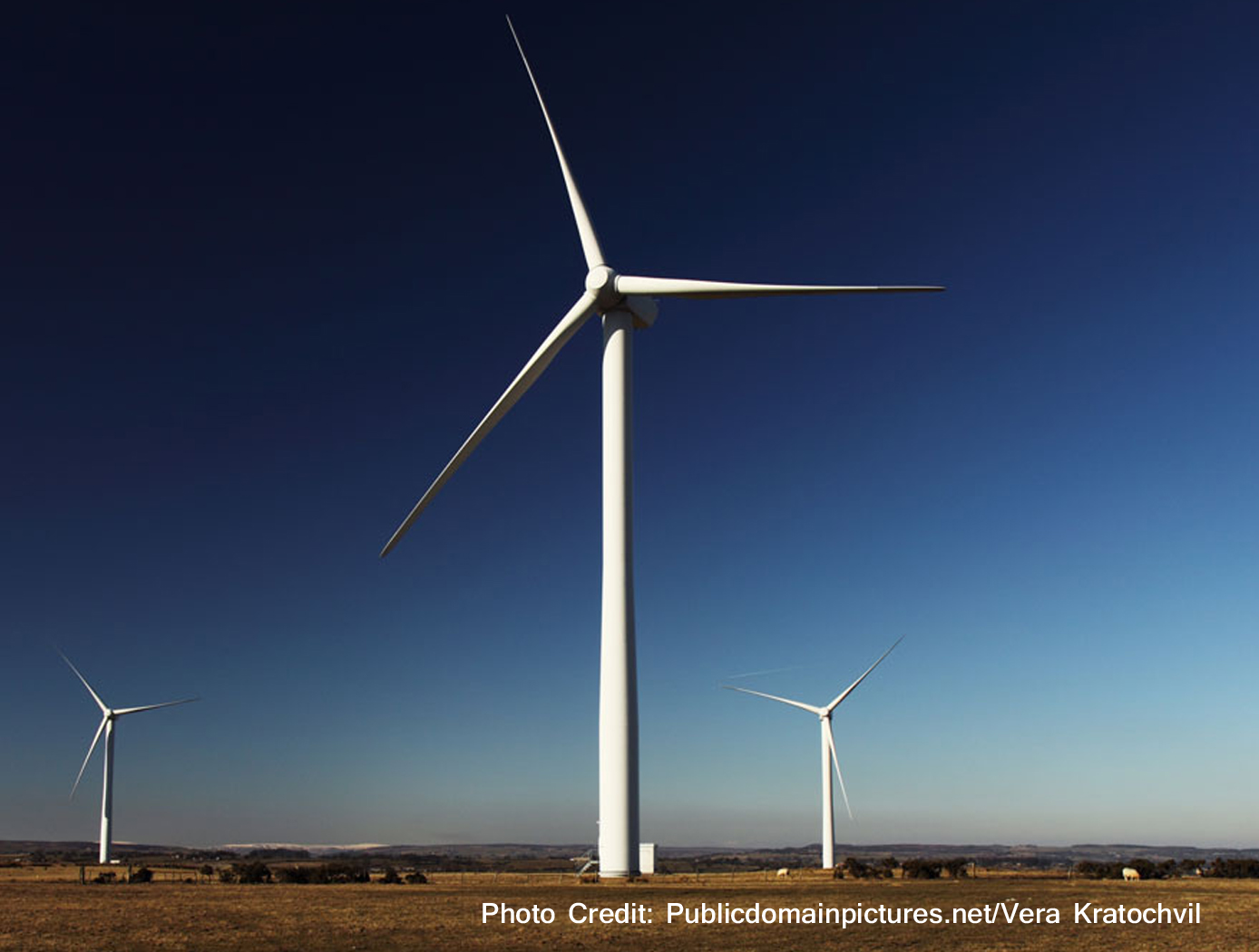
Lightning Mitigation and Damage Detection
The NASA technology can be used to protect tall structures from lightning strike damage. When a lightning leader propagates through the atmosphere in the vicinity of a tall structure, the lightning electromagnetic emissions generated from the moving electrical charge will impinge upon the tall structure before the actual charge attaches. As the lightning leader propagates closer to the tall structure, the radiated emissions at the tall structure will grow stronger. The SansEC sensor is designed to operate within the lightning radiated emission spectrum and thus is passively powered by the external oscillating magnetic field from the lightning itself. The sensor will resonate and generate its own oscillating magnetic and electric fields which have been demonstrated to influence lighting attachment and propagation.
aerospace
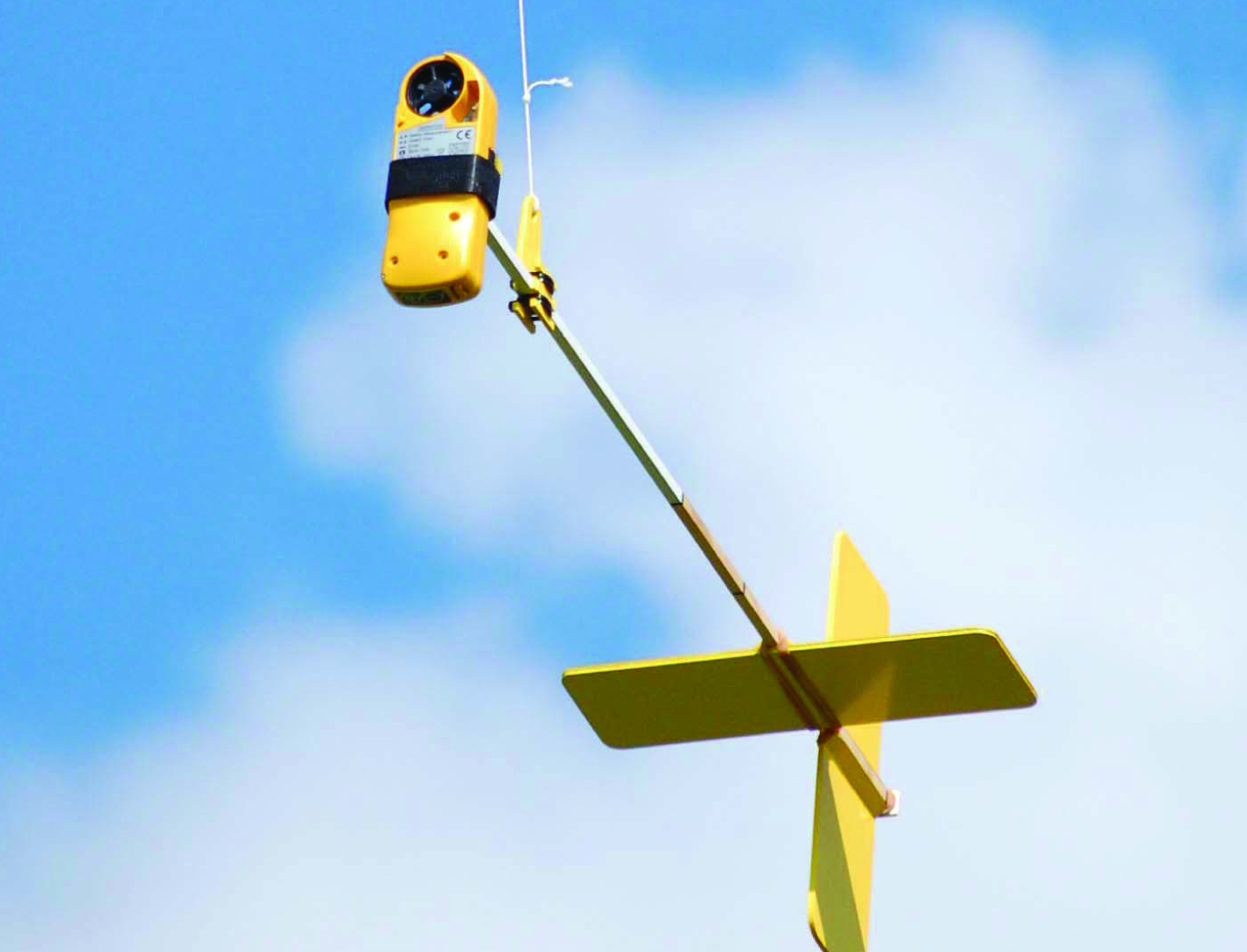
AeroPod
The AeroPods design for steadying and damping payloads includes the use of a tail boom and fin combination. It is a novel design and provides a relatively simple alternative to the traditional methods for suspending equipment from kites or blimps.
The AeroPod is superior to the traditional Picavet pulley-style suspension system for kite-flight because its light weight, simple to construct, and has no moving parts. Furthermore, the AeroPod design is advantageous to the traditional tethered blimp suspension technique where tether motion is translated directly to the sensor system because the AeroPod is free of direct motions of the tether.
sensors
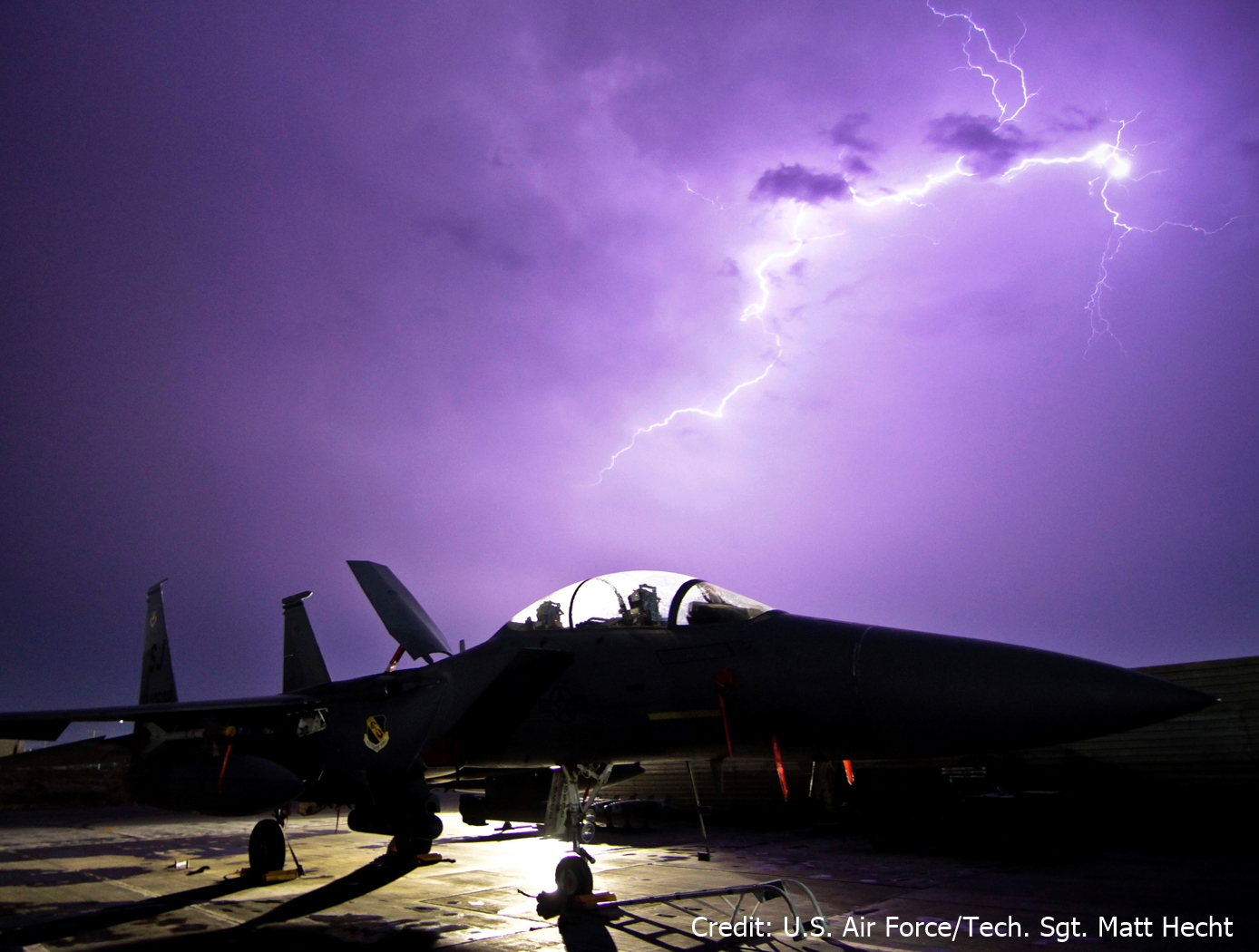
Smart Skin for Composite Aircraft
When a lightning leader propagates through the atmosphere in the vicinity of an aircraft, the lightning electromagnetic emissions generated from the moving electrical charge will radiate the aircraft surface before the actual strike to the aircraft can occur. As the lightning leader propagates closer to the aircraft, the radiated emissions at the aircraft will grow stronger. By design, the frequency bandwidth of the lightning radiated is in the range for SansEC resonance. Hence the SansEC coil will be passively powered by the external oscillating magnetic field of the lightning radiated emission. The coil will resonate and generate its own oscillating magnetic and electric fields. These fields generate so-called Lorentz forces that influence the direction and
momentum of the lightning attachment and thereby deflect/spread where the strike entry and exit points/damage occurs on the aircraft.