Search
PATENT PORTFOLIO
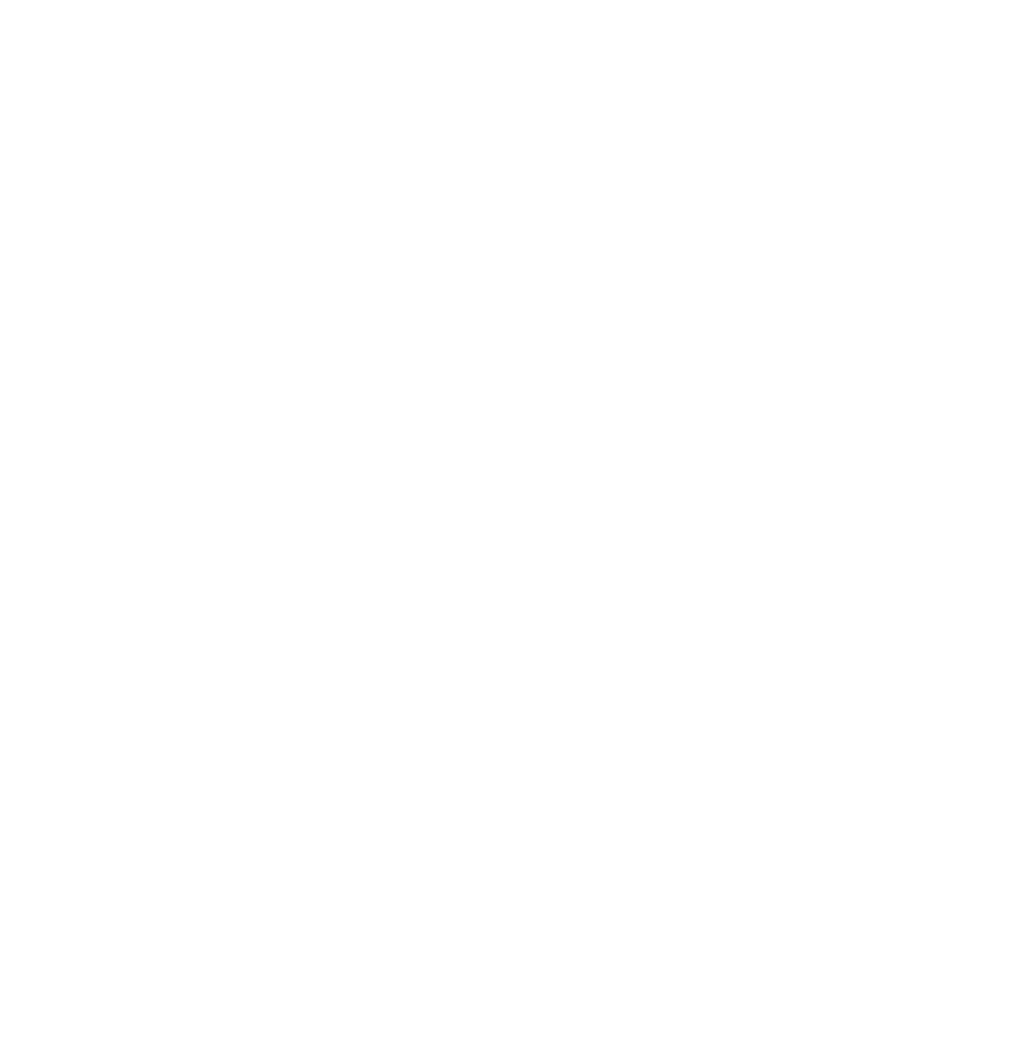
Manufacturing
NASA's portfolio of manufacturing technology is a valuable resource for companies looking to improve their capabilities and bring innovative products to market. From aerospace and defense to healthcare and beyond, NASA's manufacturing technology can help you produce high-quality products that meet the most demanding requirements. So, if you're looking to take your manufacturing to new heights, NASA's portfolio of manufacturing technology might contain your solution.
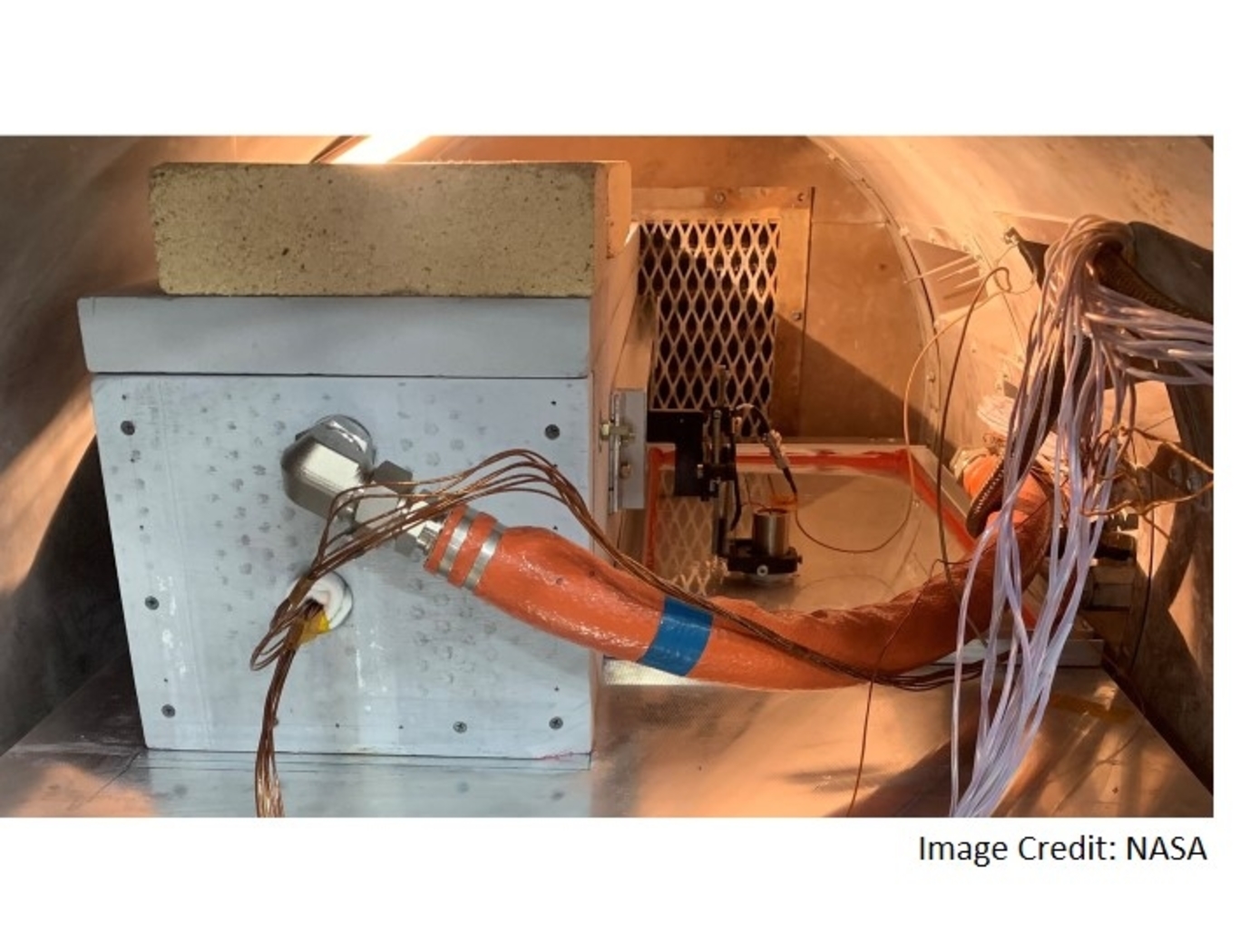
System for In-situ Defect Detection in Composites During Cure
NASA's System for In-situ Defect (e.g., porosity, fiber waviness) Detection in Composites During Cure consists of an ultrasonic portable automated C-Scan system with an attached ultrasonic contact probe. This scanner is placed inside of an insulated vessel that protects the temperature-sensitive components of the scanner. A liquid nitrogen cooling systems keeps the interior of the vessel below 38°C. A motorized X-Y raster scanner is mounted inside an unsealed cooling container made of porous insulation boards with a cantilever scanning arm protruding out of the cooling container through a slot. The cooling container that houses the X-Y raster scanner is periodically cooled using a liquid nitrogen (LN2) delivery system. Flexible bellows in the slot opening of the box minimize heat transfer between the box and the external autoclave environment. The box and scanning arm are located on a precision cast tool plate. A thin layer of ultrasonic couplant is placed between the transducer and the tool plate. The composite parts are vacuum bagged on the other side of the tool plate and inspected. The scanning system inside of the vessel is connected to the controller outside of the autoclave. The system can provide A-scan, B-scan, and C-scan images of the composite panel at multiple times during the cure process.
The in-situ system provides higher resolution data to find, characterize, and track defects during cure better than other cure monitoring techniques. In addition, this system also shows the through-thickness location of any composite manufacturing defects during cure with real-time localization and tracking. This has been demonstrated for both intentionally introduced porosity (i.e., trapped during layup) as well processing induced porosity (e.g., resulting from uneven pressure distribution on a part). The technology can be used as a non-destructive evaluation system when making composite parts in in an oven or an autoclave, including thermosets, thermoplastics, composite laminates, high-temperature resins, and ceramics.
.jpg)
Interim, In Situ Additive Manufacturing Inspection
The in situ inspection technology for additive manufacturing combines different types of cameras strategically placed around the part to monitor its properties during construction. The IR cameras collect accurate temperature data to validate thermal math models, while the visual cameras obtain highly detailed data at the exact location of the laser to build accurate, as-built geometric models. Furthermore, certain adopted techniques (e.g., single to grouped pixels comparison to avoid bad/biased pixels) reduce false positive readings.
NASA has developed and tested prototypes in both laser-sintered plastic and metal processes. The technology detected errors due to stray powder sparking and material layer lifts. Furthermore, the technology has the potential to detect anomalies in the property profile that are caused by errors due to stress, power density issues, incomplete melting, voids, incomplete fill, and layer lift-up. Three-dimensional models of the printed parts were reconstructed using only the collected data, which demonstrates the success and potential of the technology to provide a deeper understanding of the laser-metal interactions. By monitoring the print, layer by layer, in real-time, users can pause the process and make corrections to the build as needed, reducing material, energy, and time wasted in nonconforming parts.

Ultrasonic Stir Welding
Ultrasonic Stir Welding is a solid state stir welding process, meaning that the weld work piece does not melt during the welding process. The process uses a stir rod to stir the plasticized abutting surfaces of two pieces of metallic alloy that forms the weld joint. Heating is done using a specially designed induction coil. The control system has the capability to pulse the high-power ultrasonic (HPU) energy of the stir rod on and off at different rates from 1-second pulses to 60-millisecond pulses. This pulsing capability allows the stir rod to act as a mechanical device (moving and stirring plasticized nugget material) when the HPU energy is off, and allowing the energized stir rod to transfer HPU energy into the weld nugget (to reduce forces, increase stir rod life, etc.) when the HPU energy is on. The process can be used to join high-melting-temperature alloys such as titanium, Inconel, and steel.
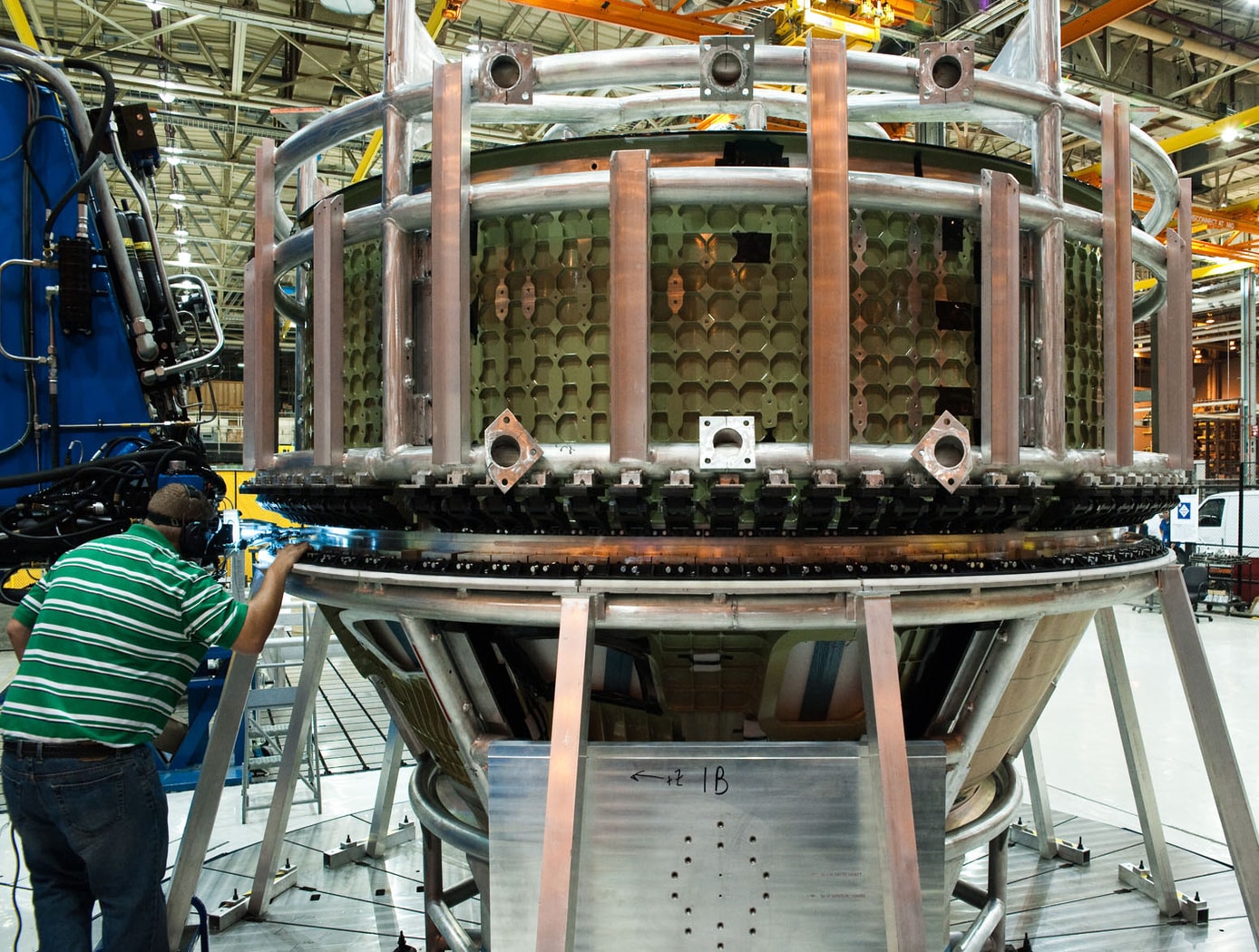
Stronger Plug for Friction Pull Plug Welding of Thick Plates
Friction Pull Plug Welding (FPPW) is the process necessary to plug the hole that is left behind as a friction stir weld (FSW) joint is completed and the pin tool of the welder retracts from the joint. FPPW involves a small, rotating part (plug) being spun and simultaneously pulled (forged) into a hole in a larger part. Much work has been done to fully understand and characterize the process and its limitations. FPPW worked very well for building large rocket sections such as the circumferential welds of the upper stages of NASA's Ares rocket, and to repair the external tank. Engineers were challenged to adapt FPPW to accommodate the thicker plates new alloy combinations of the SLS. The new hybrid plug solves the issue of the plugs snapping due to the increase torsion and moment stresses when joining thicker plates. The new hybrid plug, with a steel shank, makes FPPW more practical and robust. The new plug has been used to make space-qualified parts at NASA, and the plug welds are as strong as initial welds.
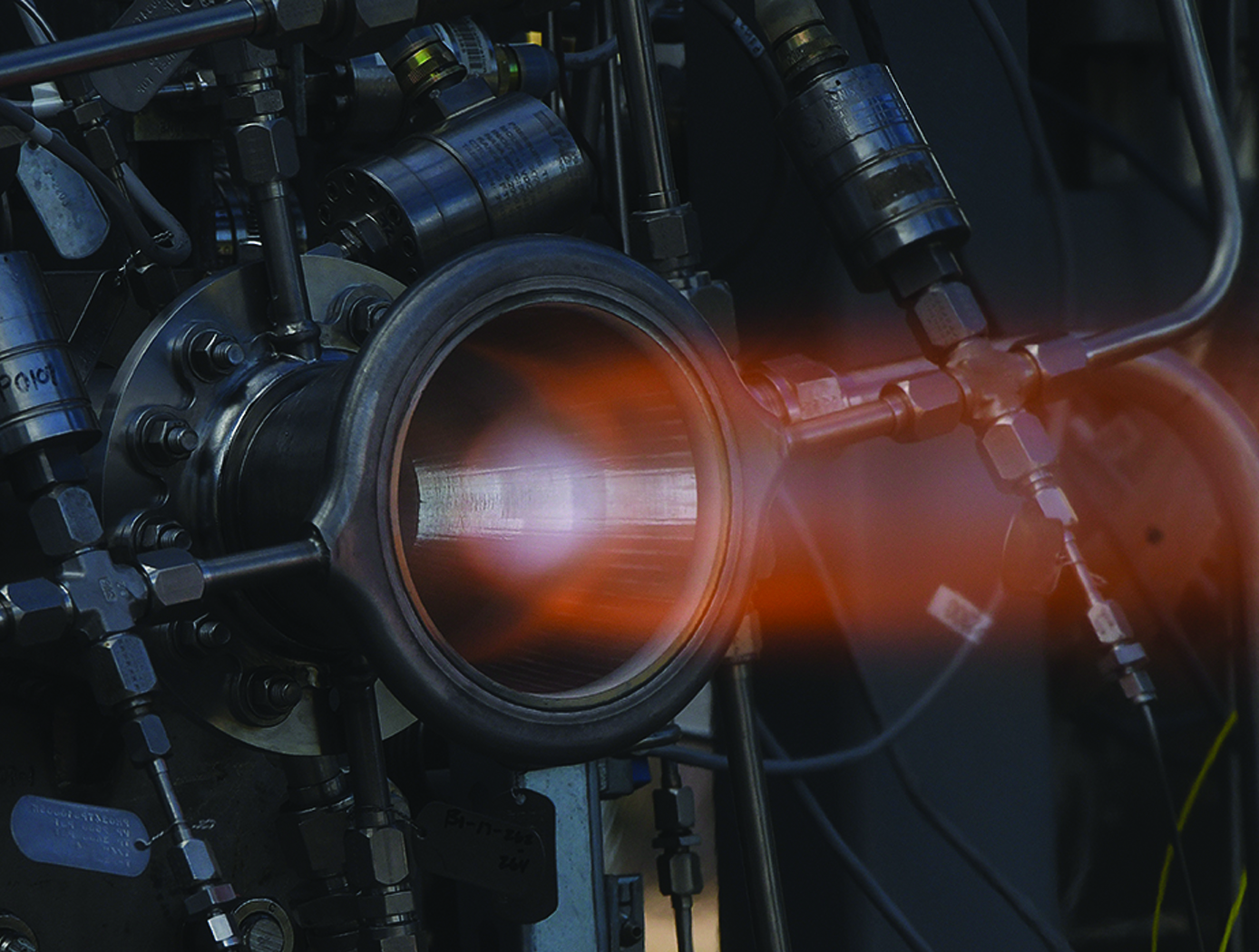
Cladding and Freeform Deposition for Coolant Channel Closeout
LWDC technology enables an improved channel wall nozzle with an outer liner that is fused to the inner liner to contain the coolant. It is an additive manufacturing technology that builds upon large-scale cladding techniques that have been used for many years in the oil and gas industry and in the repair industry for aerospace components. LWDC leverages wire freeform laser deposition to create features in place and to seal the coolant channels. It enables bimetallic components such as an internal copper liner with a superalloy jacket. LWDC begins when a fabricated liner made from one material, Material #1, is cladded with an interim Material #2 that sets up the base structure for channel slotting. A robotic and wire-based fused additive welding system creates a freeform shell on the outside of the liner. Building up from the base, the rotating weld head spools a bead of wire, closing out the coolant channels as the laser traverses circumferentially around the slotted liner. This creates a joint at the interface of the two materials that is reliable and repeatable. The LWDC wire and laser process is continued for each layer until the slotted liner is fully closed out without the need for any filler internal to the coolant channels. The micrograph on the left shows the quality of the bond at the interface of the channel edge and the closeout layer; on the right is a copper channel closed out with stainless.
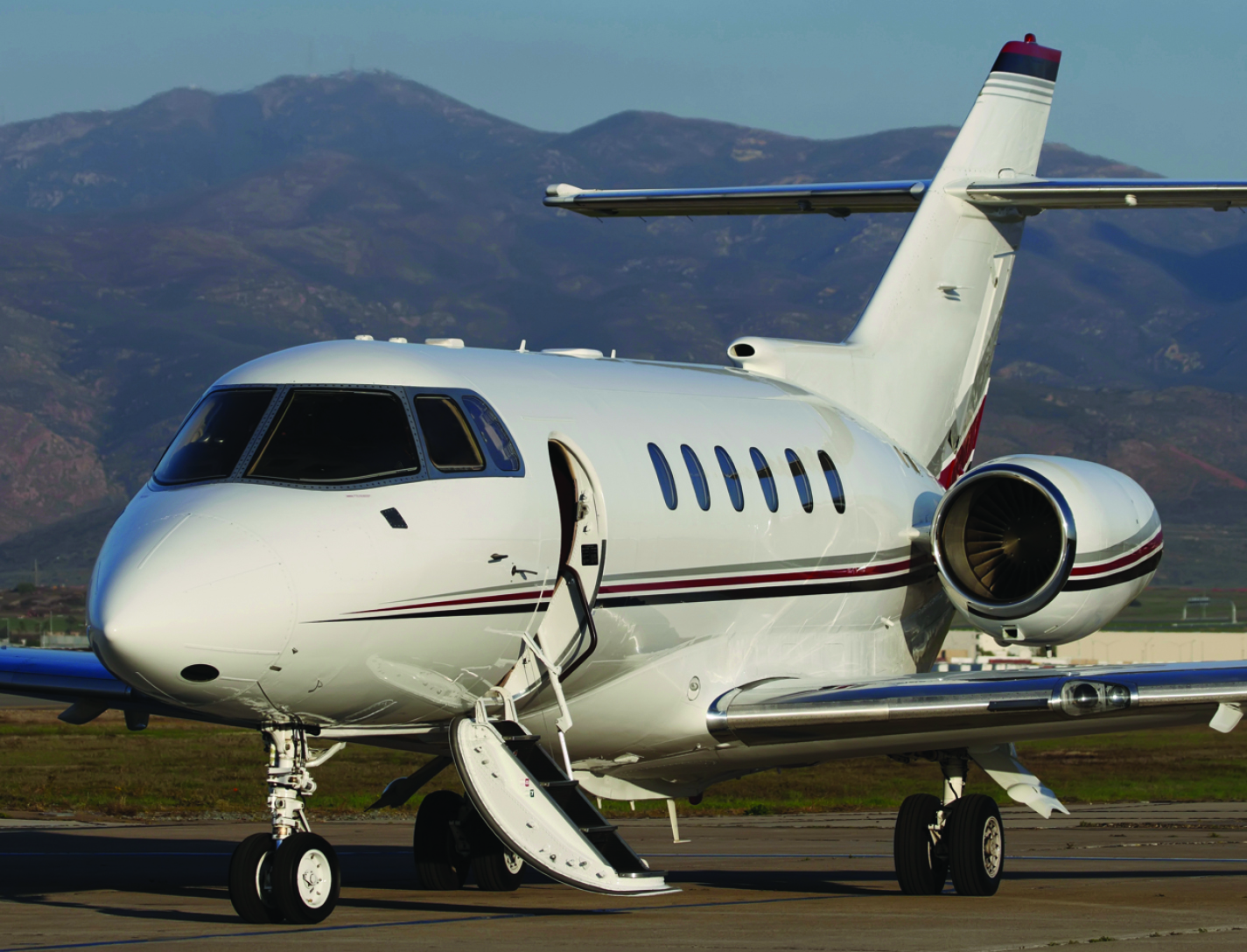
Fabrication of Fiber-Metal Laminates with Non-Autoclave Processes
The FMLs resulting from the NASA process have similar properties to traditionally produced metal/composite hybrid laminates including, as compared to either the composite or metal only structures, improved load carrying capability, lighter weight, improved stiffness, improved impact resistance and damage tolerance, and improved permeation resistance. The NASA process can be applied to various FML types, including GLARE (glass, aluminum, epoxy), and TIGR (titanium, graphite). Typical manufacturing processes are costly and complex shapes are hard to produce, whereby the NASA process enables use of these kinds of laminates without an autoclave or press, thus increasing the size that can be produced and decreasing the cost.
The resin pathways in the foils enable connection between the plies that can improve the interlaminar strength of the final part. Functionally the NASA process creates resin columns in the transverse direction of the plies. NASA is working to optimize the final properties by varying the size and distribution of the pathways.
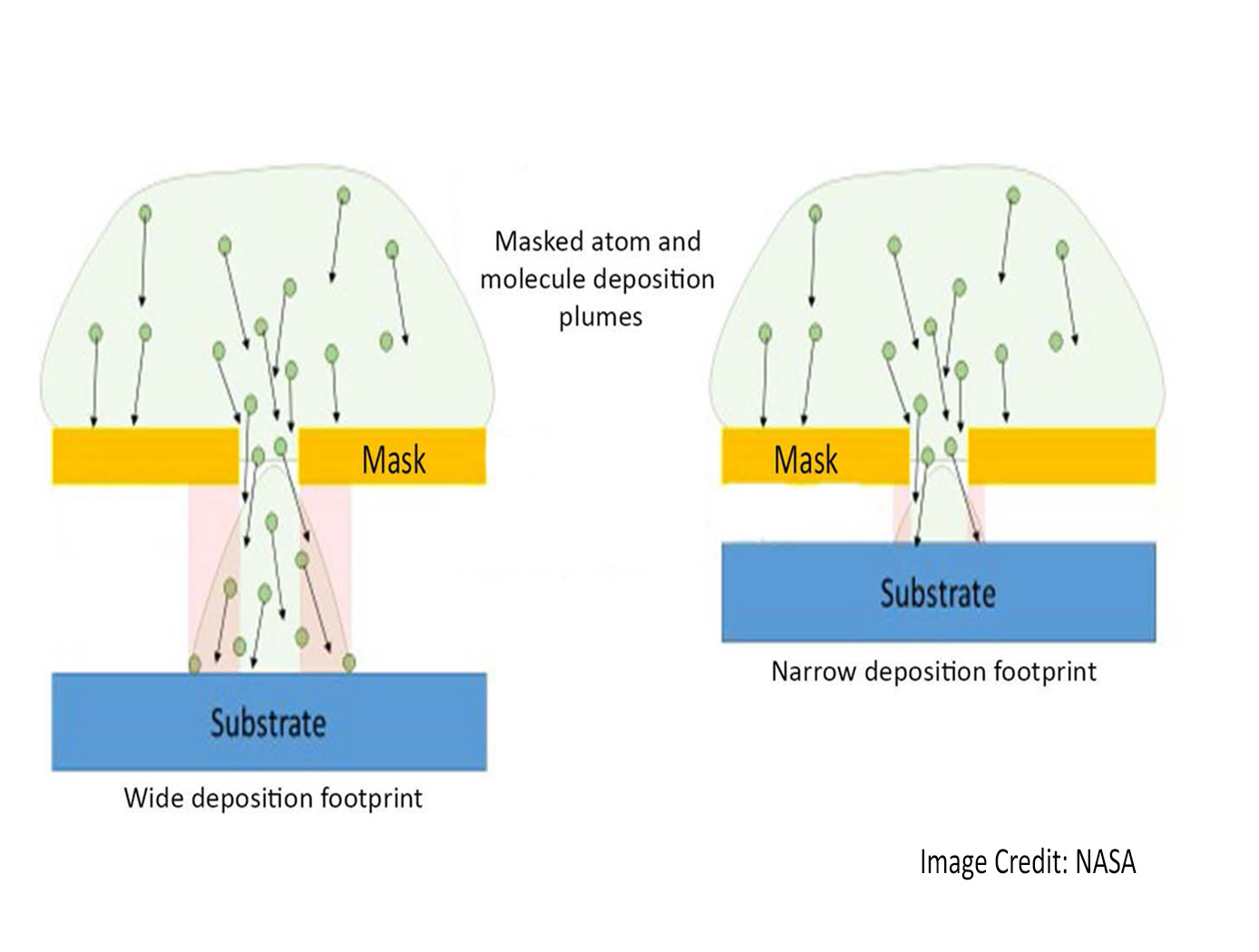
Simplified Complimentary Metal-oxide-semiconductor Manufacturing Technique
This innovation represents an alternative method to skip several of the conventional fabrication steps, using a mask designed to allow molecules in a sputtering plume to pass through openings engineered in the mask. This causes material to go where desired, without photoresist, and without selective etching, thus simplifying the process, reducing cost, chemical waste; and increasing throughput.
This method masks areas that do not need deposited material, causing local deposition instead. The shape and size are within tolerance. No etching steps, no photoresist patterning, and no metal removal is necessary. Other than planarization, selective deposition has replaced every step in the conventional process, accomplishing the same result in 8 steps instead of 19.
The technology can be easily used to fabricate custom chips and specialized sensors and devices that use the group III-V and II - VI semiconductor materials.
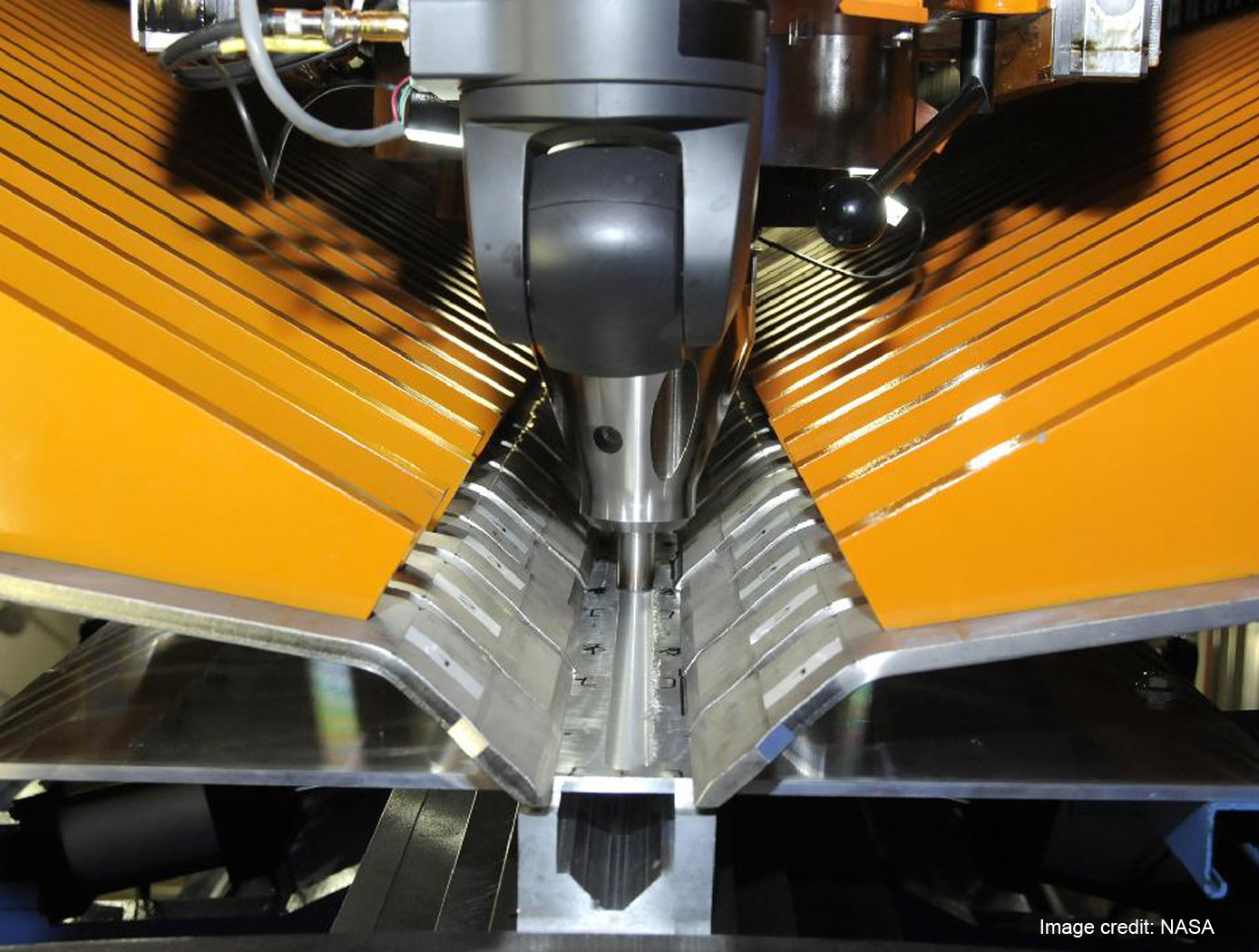
Abnormal Grain Growth Suppression in Aluminum Alloys
Heat treatment of the deformed welds is desirable in order to restore the properties of the alloy negatively affected in the weld region. In these alloys, abnormal grain growth frequently occurs in friction stir welds during solution heat treatment, and is known to degrade key materials properties, such as strength, ductility and toughness. The innovation of inserting an intermediate annealing step covered here reduces abnormal grain growth during post-welding heat treatment, thereby allowing optimum mechanical properties. This is important where Al-Li alloys (and other heat treatable alloys) are friction stir welded followed by deformation processing and high performance, high reliability structural components are required for aerospace vehicles.

Novel Overhang Support Designs for Powder-Based Electron Beam Additive Manufacturing (EBAM)
EBAM technology is capable of making full-density, functional metallic components for numerous engineering applications; the technology is particularly advantageous in the aerospace, automotive, and biomedical industries where high-value, low-volume, custom-design productions are required. A key challenge in EBAM is overcoming deformation of overhangs that are the result of severe thermal gradients generated by the poor thermal conductivity of metallic powders used in the fabrication process. Conventional support structures (Figure 1a) address the deformation challenge; however, they are bonded to the component and need to be removed in post- processing using a mechanical tool. This process is laborious, time consuming, and degrades the surface quality of the product.
The invented support design (Figure 1b) fabricates a support underneath an overhang by building the support up from the build plate and placing a support surface underneath an overhang with a certain gap (no contact with overhang). The technology deposits one or more layers of un-melted metallic powder in an elongate gap between an upper horizontal surface of the support structure and a lower surface of the overhang geometry. The support structure acts as a heat sink to enhance heat transfer and reduce the temperature and thermal gradients. Because the support structure is not connected to the part, the support structure can be removed freely without any post-processing step.
Future work will compare experimental data with simulation results in order to validate process models as well as to study process parameter effects on the thermal characteristics of the EBAM process.
View more patents